Abstract
Portal hypertension (PH) is associated with the majority of severe complications occurring in liver cirrhosis, and an increase in hepatic vascular resistance to portal blood flow underlies its pathogenesis. The reasons for this include gross structural rearrangement of the liver due to diffuse fibrosis and regenerative nodule formation, remodelling, and capillarisation of sinusoids, as well as endothelial dysfunction and impaired paracrine interaction between damaged hepatocytes, sinusoidal endothelial cells, Kupffer cells, and activated hepatic stellate cells. In this situation, intrahepatic angiogenesis is considered a compensatory mechanism aimed at portal system decompression. At the same time, newly formed vessels bypass sinusoids, so they are unable to provide oxygen and nutrients to the liver tissue, which causes disease deterioration. Angiogenesis, vascular remodelling, and endothelial dysfunction result in portosystemic shunt formation, splanchnic congestion, and hyperdynamic circulation, which leads to PH progression and the occurrence of inherent complications, in particular bleeding from oesophageal varices. Given the significant role of vascular formation and remodelling in the development of PH in cirrhosis, studying angiogenesis is important for the development of antiangiogenic therapy, which could improve associated haemodynamic disorders. To this end, various experimental models and methods, both in vitro and in vivo, of qualitative and quantitative evaluation are used.
INTRODUCTION
Portal hypertension (PH) is associated with the majority of severe complications occurring in liver cirrhosis, and an increase in hepatic vascular resistance to portal blood flow underlies its pathogenesis. The reasons for this include gross structural rearrangement of the liver due to diffuse fibrosis and regenerative nodule formation, remodelling, and capillarisation of sinusoids, as well as endothelial dysfunction and impaired paracrine interaction between damaged hepatocytes, sinusoidal endothelial cells, Kupffer cells, and activated hepatic stellate cells. In this situation, intrahepatic angiogenesis is considered a compensatory mechanism aimed at portal system decompression. At the same time, newly formed vessels bypass sinusoids, so they are unable to provide oxygen and nutrients to the liver tissue, which causes disease deterioration.1 Angiogenesis, vascular remodelling, and endothelial dysfunction result in portosystemic shunt formation, splanchnic congestion, and hyperdynamic circulation, leading to PH progression and the occurrence of inherent complications, in particular bleeding from oesophageal varices.2 Given the significant role of vascular formation and remodelling in the development of PH in cirrhosis, studying angiogenesis is important for the development of antiangiogenic therapy, which could improve associated haemodynamic disorders.3 To this end, various experimental models and methods, both in vitro and in vivo, of qualitative and quantitative evaluation are used.4
INTRAHEPATIC ANGIOGENESIS ASSAYS
Appearing simultaneously with fibrosis, intrahepatic angiogenesis causes abnormal angioarchitecture formation characteristic of cirrhosis, which is the endpoint of the disease. Therefore, evaluation of intrahepatic angiogenesis is necessary to assess disease progression and search for therapeutic targets (Table 1).5
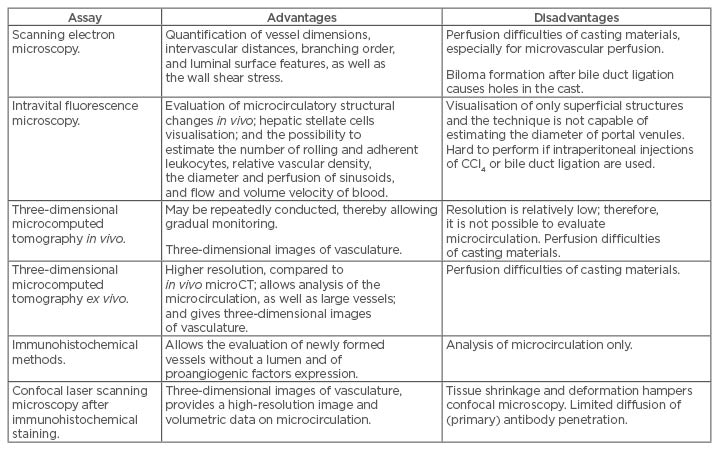
Table 1: Advantages and disadvantages of intrahepatic angiogenesis assays.
Scanning Electron Microscopy
Scanning electron microscopy is the traditional method for studying the three-dimensional (3D) structure of microcirculation. It is visualised by an electron beam after intravascular injection of coloured gelatin, latex, or plastic casting material followed by tissue clearing or corrosion. Unlike tissue sections, this approach makes it possible to not only quantify vessel dimensions, intervascular distances, branching order, and luminal surface features, but also to mathematically calculate the wall shear stress.6 Using scanning electron microscopy in rats with cirrhosis caused by subcutaneous injections of carbon tetrachloride (CCl4) (0.3 mL 50% CCl4 diluted with oil per 100 g of body weight twice a week for 3 months), it was determined that the number of sinusoidal endothelial fenestrae decreased and the sinusoids within the regenerative nodules surrounded by fibrous septa were narrow.7 In mice with biliary cirrhosis, this technique allowed for the identification of numerous blindly terminating and chaotically located sinusoids, as well as large portosystemic collaterals bypassing them and shunting blood towards the hepatic veins. The disadvantage of bile duct ligation is biloma formation, causing irregular saccular deformation of sinusoids, which presents as holes in the cast. Moreover, limitations of the casting technique include perfusion difficulties, especially for microvascular perfusion, and bloating of the sinusoids due to the injection pressure. This makes it difficult to perform a more thorough morphometric analysis of the hepatic microvasculature.8
Intravital Fluorescence Microscopy
Intravital fluorescence microscopy is necessary for the intravital evaluation of structural changes at the microcirculatory level in experimental cirrhosis. For this purpose, different models of cirrhosis may be used, such as bile duct ligation and subcutaneous or intraperitoneal administration of CCl4.9 After performing midline and subcostal incisions, the hepatic ligaments were dissected, and the left liver lobe was placed on a fixed plate to minimise respiratory movements. A fluorescent dye was injected into the jugular or tail vein, and, while tissue contrast is increased, liver microcirculation was analysed with a fluorescent microscope.
Intravital fluorescence microscopy makes it possible to visualise hepatic stellate cells due to autofluorescence of vitamin A; estimate the number of rolling and adherent leukocytes; and measure relative vascular density, the diameter and perfusion of sinusoids, and flow and volume velocity of blood.10,11
The maximal number and diameters of portosystemic shunts were observed 3 weeks after bile duct ligation and 12 weeks after the first administration of CCl4 (0.1 mL 50% oil solution per 100 g of body weight subcutaneously twice a week for 4 months; 5% alcohol was added to drinking water).10 Fibrosis and the activated hepatic stellate cells were located in periportal areas in biliary cirrhosis and in pericentral areas in CCl4-induced cirrhosis. The sinusoids in these regions became narrow, whereas the distance between them increased.10
Although subcutaneous CCl4 administration can induce necrosis at the site of injection and should be carried out for 16 weeks, this route of administration is preferable for intravital fluorescence microscopy.10,12 In contrast to intraperitoneal injections or bile duct ligation, subcutaneous CCl4 administration does not cause adhesions between the liver and the neighbouring organs that can later limit the possibility of carrying out in vivo studies in the abdominal cavity. Despite the fact that the oral application of CCl4 enhances mortality rate,13 a modification of this technique by Proctor and Chatamra14 has been developed. The dose was individualised according to weight gain/loss of the animal in response to the previous dose. Short inhalation cycles of CCl4 two or three times weekly should also be considered to avoid extrahepatic damage.15
The major drawback of intravital fluorescence microscopy is that it can only visualise superficial structures; therefore, it does not give an opportunity to estimate the diameter of portal venules. This may be due to the displacement of portal tracts deeper into the tissue as a result of pericentral fibrosis.
Three-Dimensional Microcomputed Tomography
Microcomputed tomography (microCT) provides high-resolution 3D images that are composed of two-dimensional (2D) trans-axial projections, or ‘slices’, of a target object. For live animal imaging, the slices are obtained by rotating the emitter and detector. To evaluate microcirculation, ex vivo microCT requires vascular casting with contrast agents, such as microfil MV-122 and BaSO4/gelatin, whereas in vivo microCT can be performed with iodinated monomer-based bolus or lipid emulsion-based blood-pool contrast agents.16
Intravital microCT is performed with a dual-energy flat-panel microCT scanner before and immediately after intravenous injection of 100 μl of specially optimised iodine-based contrast agent eXIA™160XL (Binitio Biomedical Inc., Ottawa, Canada). A Feldkamp-type algorithm is used to reconstruct 2D images into 3D with a voxel size of 35x35x35 μm. The relative blood volume value determination is based on the mean brightness of the liver tissue after a contrast agent injection. The value correlates with the number of angiogenic vessels. In this way, a statistically significant increase in the relative blood volume was observed in the murine cirrhotic liver 6 weeks after the first intraperitoneal administration of CCl4 (0.06 mL 50% oil solution per 100 g of body weight twice a week for 6 weeks) and 2 weeks after bile duct ligation. The advantage of the technique is that it can be conducted repeatedly, thereby allowing gradual monitoring of the process.
The merit of ex vivo microCT is the higher resolution image obtained.17,18 It was used in different models of cirrhosis in mice and rats. In its classical version, the inferior vena cava is crossed above the diaphragm, and the radiopaque lead oxide diluted in a liquid silicone polymer (microfil) was injected into the portal vein at the rate of 8–10 mL/min and at a pressure of 10–12 mmHg; or alternatively, injection into the heart at a pressure not higher than that of the artery, this is carried out using an automatic pump. The specimens are kept at 4°C for 12 hours. Subsequently, the liver is taken, cut into lobes, fixed in formalin, and then dehydrated in increasing concentrations of a glycerol aqueous solution at 24-hour intervals. Using a microCT scanner and the special computer processing algorithm, 3D images of the intrahepatic microvasculature are obtained and then analysed using software.19
MicroCT ex vivo makes it possible to estimate the ratio of vascular volume to total liver volume and enables precise analysis of the branching of medium and large hepatic vessels. They are detected in the liver, particularly at the periphery, 6 weeks after the first intraperitoneal injection of CCl4 (0.06 mL 50% oil solution per 100 g of body weight twice a week for 6 weeks) and 21 days after bile duct ligation. By Week 4 of biliary cirrhosis development, the vascular volume increased one and a half times and the number of branches doubled,20 which reflected the severity of angiogenesis.21
The limitations of the technique include perfusion difficulties, especially for microcirculatory perfusion, and the reactivity of casting resins with other chemical compounds and surrounding tissue. Moreover, dual casting, which is necessary for contrast-based differentiation between venous and arterial systems, is not possible. This is due to the presence of shunts between the hepatic arterioles and portal venules, functioning as a one-way valve that allows blood to flow only from the arterial to venous system.22 To overcome this shortcoming, Peeters et al.23 sequentially injected yellow or blue contrast agents, PU4ii, into the abdominal aorta or portal vein, respectively, after clamping the thoracic aorta and renal arteries. In order to prevent damage to the microvessels by the pumped substance, a polyethylene drainage tube was installed into the inferior vena cava through the right atrium. The thoracic section of the inferior vena cava, the abdominal aorta, and the portal vein were clamped to eliminate leakage of the substance during polymerisation. After 72 hours, the casted liver was macerated using a 25% potassium hydroxide bath for 5 days. The vascular replica was then flushed with distilled water and laid to dry under a vented hood for a further 5 days. MicroCT with 3D reconstruction was performed with a resolution of 1.89 μm for microcirculation and 40 μm for larger vessels. To morphologically analyse microcirculation, a sample with the dimensions 350х350х200 μm was virtually dissected in between portal triads. The average radius of the sinusoidal vessels, branch length, tortuosity, and porosity (the total sinusoidal volume divided by the volume of its envelope) of the vascular network was assessed with the in-house developed software. Using this technique in animals with macronodular cirrhosis induced by thioacetamide (first, 0.03% thioacetamide was added to drinking water, and then the concentration was adapted every week for 18 weeks to keep body weight within a 250–300 g range), these authors have identified the compression of the hepatic venules and an increase in the diameter of the hepatic artery.24
Immunohistochemical Methods
Intravascular injection of contrast agents only revealed the structure of functioning vessels. Immunohistochemical staining of tissue sections is used for a more accurate evaluation of the newly formed vessels, including the nascent capillaries without a lumen. The most common specific markers are vascular endothelial growth factor (VEGF) and membrane proteins CD31 and CD34 for endothelial cell detection. In particular, VEGF and angiopoietin-1 expression shows an increase in intrahepatic vascular density in rats with biliary cirrhosis.21 Immunofluorescence is a variant of immunohistochemical staining. It requires the use of secondary antibodies such as streptavidin conjugated with carbocyanine CY2.25
Confocal laser scanning microscopy after immunohistochemical staining makes it possible to study 3D structures with a resolution of up to 0.2 μm. This technique is based on improved protocols for the chemical purification of samples allowing a dye and photons to penetrate deeper into tissue before and after immunohistochemistry.26,27 Subsequent confocal laser scanning provides detailed volumetric data on microcirculation at a voxel size of 0.63×0.63×1.40 μm. The data are processed by a specially developed software (DeLiver). In a study involving rats with thioacetamide-induced cirrhosis, a decrease in mean radius and porosity as well as an increase in tortuosity and length of sinusoids were determined using this technique.24
EXTRAHEPATIC ANGIOGENESIS ASSAYS
Violations of organ and systemic haemodynamics and portosystemic collateral circulation in PH begin with splanchnic vasodilation and neovascularisation, which are caused by hypoxia of the small intestinal mucosa with the participation of proinflammatory cytokines, chemokines, and angiogenic factors such as VEGF, platelet-derived growth factor, phosphatidylinositol glycan anchor biosynthesis class F, and others. Portosystemic shunts become clinically significant when in the presence of gastro-oesophageal varices, the rupture of which leads to life-threatening bleeding. It was traditionally thought that these varices are formed when increased portal pressure causes the opening of pre-existing vessels in the areas of embryonic communication between the portal and systemic circulation. This paradigm was challenged by Fernandez et al.,28 who first reported that portosystemic collaterals in PH are formed due to active angiogenesis (Table 2).
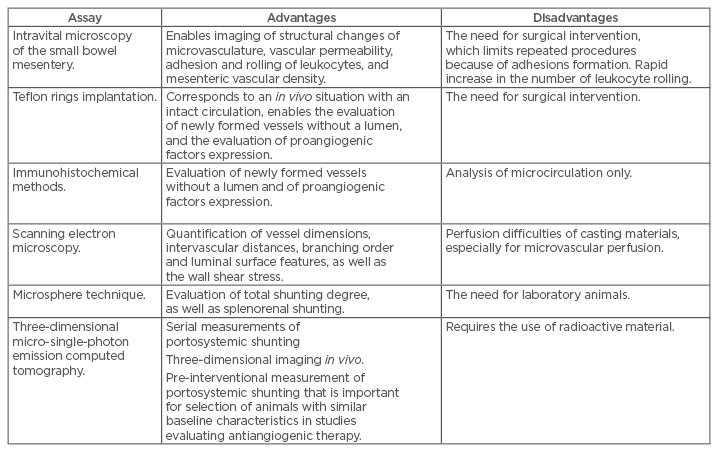
Table 2: Advantages and disadvantages of extrahepatic angiogenesis and portosystemic shunting assays.
Intravital Microscopy of The Small Bowel Mesentery
Intravital microscopy enables imaging of structural changes of microvasculature, vascular permeability, and mesenteric vascular density, which characterises splanchnic angiogenesis. After performing a midline laparotomy, a small intestinal loop was exteriorised, placed on a heated plexiglas plate, and continuously superfused with an Earle’s balanced salt solution to prevent dehydration. Observations were carried out with the Axiotech Vario 100HD microscope (Carl Zeiss AG, Oberkochen, Germany) equipped with water immersion objectives (x10 and x40). The obtained image was recorded for the subsequent computer analysis. This enabled study of all the types of mesenteric microvessels and calculation of their density, which is defined as the ratio of a vessel’s length to the area it occupies.29 Epifluorescence microscopy is used to measure vascular permeability. After the selection of a venular segment with a diameter of 20–40 mm and an unbranched length of about 150 mm, fluorescent isothiocyanate-bovine serum albumin was injected intravenously. As the intraluminal grey scale value fell, the perivascular grey scale value rose when the fluorescent isothiocyanate-bovine serum albumin molecule leaked through the vascular wall, quantified using black and white image and taking black for 0 and white for 255.
Intravital microscopy revealed an increased vascular density and gross disturbance of the mesenteric microcirculation in rats with PH induced by partial portal vein ligation (PPVL) and in rats with biliary cirrhosis. Moreover, the changes in the latter were more significant, which may be due to the time required for cirrhosis development. In addition, vascular permeability was significantly increased in these animals, in contrast to permeability in animals with extrahepatic PH. This is explained by the higher levels of endothelial nitric oxide synthases and VEGF.30
In addition to the aforementioned uses, intravital microscopy is used to quantify adhesion and rolling of leukocytes, a well-known hallmark of inflammation, which in turn leads to angiogenesis. In particular, in rats with cirrhosis induced by CCl4 (0.04 mL administered intragastrically and increased weekly in increments of 0.04 mL to a maximum dose of 0.4 mL; 35 mg/100 mL phenobarbital was added to drinking water once 2 weeks before the first administration of CCl4), the index of leukocyte-endothelial interaction was increased in the microcirculation of the liver and small bowel mesentery.31 The disadvantage of this method is the need for surgical intervention and extraction of the mesentery from the abdominal cavity. This causes a rapid and pronounced increase in the number of leukocyte rolling in response to partial degranulation of perivascular mast cells and endothelium expression of P-selectin in a matter of minutes.32
The Requirements for the Analysis of Microcirculation Images Obtained with Intravital Microscopy
Intravital microscopy requires video recording for the analysis of microvasculature. It is recommended that the following requirements are met:33
- At least three, or preferably five, arbitrary regions of microcirculation should be included in the analysis.
- Optical magnification should be x10 for microcirculation imaging in small laboratory animals.
- It is necessary to avoid pressure artifacts occurring when a microscope objective contacts the region of interest; excess pressure applied to the area may collapse the microcirculation and stop venous blood flow.
- Video images should be stored in full size without compression to the form of DV-AVI files in order to provide the possibility of computer frame-by-frame analysis. The optimal video recording time is 20 seconds.
A report on the analysis of images obtained with intravital microscopy must include the following parameters calculated for all vessels and capillaries separately:
- Total vascular density and perfused vessel density, which are calculated as the ratio of total vessel length to image area.
- Proportion of perfused vessels expressed as a percentage.
- Microvascular flow index.
- Heterogeneity index, which is calculated as the ratio of the difference between the maximum and minimum blood flow velocity to its average velocity in the five selected areas of an image.
Calculations can be made directly by the researcher using semiquantitative scales for visual evaluation.34,35 For a more accurate analysis of the required parameters, the CapImage software is used. It was specially developed for intravital microscopy.36
In Vivo Evaluation of Angiogenesis in the Small Bowel Mesentery by Implantation of Teflon Rings
Implantation of Teflon rings is another technique for intravital evaluation of angiogenesis of the small bowel mesentery in PH. The rings have a diameter of 7 mm, a height of 3 mm, and an internal diameter of 5 mm. The rings are placed into polyester mesh bags and filled with a mixture of bovine Type I collagen and bovine serum albumin. After performing a midline incision and PPVL, the rings were implanted between the two mesenteric membranes and fixed with single sutures in rats. After 16 days, the rats were euthanised, the implant was removed, fixed in a 4% formaline, and paraffinised. Then, 3-μm-thick tissue sections were prepared. The tissue was stained for further video morphometry and vascular density calculation. This technique makes it possible to determine the number of vessels and the mechanisms of their formation.37
Immunofluorescence Assay
Vascular network imaging can be carried out by immunological reaction of fluorescent antibodies with membrane proteins of endothelial cells. Anti-CD31 and anti-VEGF antibodies are most often used for these purposes.38,39 The small intestinal wall or its mesentery was washed in sodium phosphate buffer, dried on gelatin-coated slides, and fixed in 100% methanol at -20°C for 30 minutes. The sections were then incubated with the corresponding primary murine anti-rat antibodies at 4°C for 12 hours. Streptavidin conjugated with carbocyanine CY2 was used as a secondary antibody. It was applied at room temperature and held for 1 hour. The image obtained after fluorescence microscopy may be analysed using ImageJ software.40 An alternative technique consists of the fixation of frozen sections in acetone at -20°C for 10 minutes. After which, the tissue is blocked with 5% bovine serum albumin solution for 45 minutes.41 Besides CD31, endothelial cell identification is possible with the use of BSI-lectin. Perivascular cell markers include Neural/glial antigen 2, desmin, α-smooth muscle actin, platelet-derived growth factor receptor-β, and Class III β-tubulin.42
Immunohistochemical Staining
Immunohistochemical staining is performed to study not only intrahepatic but also extrahepatic angiogenesis, including angiogenesis in the small bowel mesentery and the gastric wall. The presence of angiogenesis in the small bowel mesentery was confirmed by numerous experimental studies in laboratory animals with different PH models.43
Tissue oxidative stress, which occurs in PH, aggravates the pathophysiological changes that occur in the gastric wall. The oxidative stress is detected by the reaction of antibodies with metabolites that arise during free radical oxidation, such as nitrotirozine. In particular, an increased expression of endothelial nitric oxide synthases, VEGF, and nitrotyrosine was found in the gastric wall of rats with prehepatic PH induced by PPVL, indicating the presence of stimuli for further development of collateral circulation.44
Aperio45 or the CAIMAN algorithm46 may be used to calculate the number of vessels on images of immunohistochemically stained samples. The programme called AngioPath can quantify microvessels and determine the size and shape of all vessels as well as each vessel individually. As such, it is an important tool for characterising angiogenesis.47
Scanning Electron Microscopy
Scanning electron microscopy of vascular casts is helpful for imaging of the splanchnic vascular network’s 3D structure with its subsequent quantitative analysis. The technique was used in animals with PPVL and biliary cirrhosis and revealed the presence of newly formed tortuous vessels serving as shunts between the branches of the inferior vena cava and the portal vein. In addition, holes were found in the walls of some capillaries, serving as a sign of intussusceptive angiogenesis, which is one of the two known types of microvessel growth.8
ASSESSMENT OF PORTOSYSTEMIC SHUNTING
Portosystemic Shunting Assay Using Microspheres
In 1981, Chojkier and Groszmann48 proposed to use 51Cr-labelled microspheres to assess the degree of portosystemic shunting. The modification of Chojkier and Groszmann’s48 technique through the use of colour polystyrene fluorescent microspheres has become widespread at the present time, because this technique excludes any contact with the dangerous radioactive material and maintains accuracy.49
Approximately 30,000 yellow microspheres (15 μm in diameter) are slowly injected into the spleen. An injection of microspheres of a different colour into the ileocolic vein should be completed for a more detailed haemodynamic assessment of total shunting from the splanchnic area.50 The liver and lungs of the animal models were removed and placed in centrifuge tubes. Approximately 3,000 blue microspheres were added as an internal control. The tissue was digested in unilocular potassium hydroxide at 60°C for 12 hours and then sonicated. After centrifugation, the supernatant was removed and the pellets were washed once in 10% Triton X-100 solution and twice in acidified ethanol. The precipitate containing microspheres was dried for 12 hours, diluted in acidified Cellosolve™ acetate (The Dow Chemical Company, Midland, Michigan, USA), and the number of microspheres was counted using a spectrophotometer. A haemocytometer and an epifluorescent microscope are also adequate for this procedure.51 The degree of portosystemic shunting is calculated as the ratio of the number of pulmonary microspheres to their sum in the lungs and liver.52
The microsphere technique confirmed that portosystemic collaterals had started forming in rats with PH 2 days after PPVL and became fully developed on Day 7 post PPVL.28 At the same time, portosystemic collaterals developed later in rats with biliary cirrhosis. Therefore, it is expedient to evaluate them 1 month after bile duct ligation.53
Three-Dimensional Micro-Single-Photon Emission Computed Tomography
Since a significant disadvantage of the microsphere method is the need to use laboratory animals, micro-single-photon emission computed tomography (3D micro-SPECT) with technetium (99mTc) macro aggregated albumin was developed as an alternative. It provides a possibility of conducting serial measurements of portosystemic shunting at different time points after the creation of a model. 99mTc macro aggregated albumin particles are injected into the splenic pulp; accumulation of the particles in the liver and lungs is determined by using colour scales and computer processing.
3D micro-SPECT was used in mice with PPVL and biliary cirrhosis. The results correlated with the results obtained by using 51Cr-labelled microspheres, and there were no lethality or changes in animals’ behaviour after its reusing on the 8th, 12th, and 15th day after the model creation.54
FUTURE INSIGHTS INTO ANTIANGIOGENIC THERAPY IN PORTAL HYPERTENSION
Advances in understanding the pathogenesis of PH in cirrhosis stimulated the development of new methods for its pharmacotherapy. β-adrenoblockers are not recommended for use during the subclinical stage of the disease, while aetiotropic and pathogenetic treatment is reasonable.55 It may affect, for example, fibro and angiogenesis in the liver (tyrosine kinase inhibitors56 and statins57), as well as angiogenesis that underlies portosystemic shunts formation (tyrosine kinase inhibitors,58 somatostatin and its analogs,59 and N-acetylcysteine,53 among others). This approach, as a part of the complex correction of pathophysiological mechanism contributing to the development of PH, may be the key to success in preventing related complications.
CONCLUSION
The application of modern techniques for studying angiogenesis in experimental research made it possible to establish the important role of new vessel formation in PH pathogenesis. Despite the initially compensatory function, angiogenesis leads to the progression of PH inherent haemodynamic disturbances. The development of antiangiogenic therapy, which would be selectively directed to unusually growing newly formed vessels, may be a promising direction in the treatment of PH and associated complications.