Abstract
Wheat intolerance is a common problem for certain individuals. A gluten-free diet is the only option for people with wheat-associated disorders (WAD) to manage their condition. The elimination of immunogenic proteins from the wheat is the most appropriate approach to ameliorate the symptoms of affected individuals, while also meeting their nutritional requirements. RNA interference technology can be exploited to silence the expression of gliadins to produce a wheat variety lacking the immunogenic proteins associated with WAD, but there are challenges before implementation of transgenic varieties in the market will occur. This review is focussed on RNA interference approaches acquired to produce wheat that patients with different WAD can tolerate. The authors also discuss the advantages and disadvantages of current omics approaches that are being used to validate the food safety issues related to the applicability and clinical relevance of genetically modified wheat.
INTRODUCTION
Wheat is the causal factor for a number of diseases, including coeliac disease (CD), wheat-dependent exercise-induced anaphylaxis (WDEIA), wheat allergy, dermatitis herpetiformis, and non-coeliac gluten sensitivity (NCGS). The literature is scarce about the prevalence of most wheat-associated disorders (WAD) except for CD. Recently, the prevalence of wheat allergy in a birth cohort (10 and 11 year olds) was reported as 0.48% in the UK,1 whereas prevalence was measured between 1.2 and 75.3 per 100,000 people for dermatitis herpetiformis.2-8 Research conducted in the USA reported the prevalence of NCGS to be 6.0% in a hospital-based study,9 but another study reported a 0.55% prevalence of NCGS in the general population of the USA.10 CD is the most studied and prevalent among all wheat-related diseases. CD was first reported to have a prevalence of 1:184 in an Italian school-aged paediatric population.11 Prevalence of CD varies from 0.006% to 5.600% in different populations across the world.12-20 The Saharawi population of Africa accounts for 5.6% of CD cases. CD is considered the most prevalent wheat-related disease across the world.21 In addition, the prevalence of CD is around 11% in the Type 1 diabetes mellitus population in India.22 CD is also the cause of other diverse health problems23,24 because CD is an autoimmune disorder of the small intestine that leads to destruction of intestinal villi as a consequence of inflammation.25,26 Though there are some genetic and environmental factors associated with disease,27-31 wheat gluten (wheat protein) remains the antigenic trigger of CD, and its withdrawal from the diet improves the clinical conditions of CD patients. The extent of gluten intake is strongly associated with the severity and prevalence of CD.20
Even after decades of research, there is no successful treatment available as an alternative to a gluten-free diet (GFD). Strict lifelong adherence to a GFD always remains a challenge for CD patients and even occasional ingestion of gluten-containing food facilitates reoccurrence of gluten-induced inflammation. Moreover, some CD patients never recover from symptoms, even after remaining on a GFD for a long time, due to intestinal dysbiosis.32 Although a GFD is beneficial for patients, some clinical reports have shown that its effectiveness is compromised in malnourished patients.33 Therefore, exclusion of wheat from the diet may lead to secondary problems that may persist, along with an altered intestinal microbiota.34
Currently, trials of ongoing therapies in CD patients are still evoking different opinions with regard to eliciting a beneficial response. Thus, a wheat variety lacking the immunogenic components of gluten may prove to be beneficial for patients with this condition. Similarly, RNA interference (RNAi) is being used by the scientific community to produce a wheat variety devoid of immunogenic proteins. Other studies have used metabolomics techniques as markers of biosafety for crops, with an aim to elucidate the effect of fertilisers on the metabolome of plants. Although metabolomics in this field is in its infancy, this review elucidates the use of metabolomics in the development and validation of safety measures with regard to genetically modified (GM) plants. Hence, metabolomic approaches have the potential of elucidating better safety measures from a health point of view.
IMMUNOGENIC GLUTEN PEPTIDES: THE CAUSAL FACTOR FOR COELIAC DISEASE
Gluten is the protein component of wheat that contains α-gliadins, γ-gliadins, and glutenins as immunogenic components, and ω5-gliadins as the allergen component. Human proteolytic enzymes cannot effectively digest gluten proteins,35 and these proteins have been reported to possess differential immune targets that make them immunotoxic by nature. During proteolytic digestion in the intestine, immunogenic proline and glutamine-rich gluten polypeptides are produced that can stimulate T cells.36 These peptides are resistant to further hydrolysis due to enrichment of proline residues in the amino acid sequences.37 The peptides 33-mer and 26-mer, derived from α-gliadin and γ-gliadin, respectively, subsequently trigger immunological responses in the intestines of CD patients.25,38-40 Peptide 31−49 of α-gliadin was reported to be a potent activator of innate immune processes in the mucosa of CD patients when tested on T cell lines established from the CD intestinal mucosa.41 Another similar peptide of α-gliadin was tested in a Caco-2 cell line model and was reported to be resistant to digestive enzymes, with the potential to penetrate across a Caco-2 monolayer.42 The immunogenic potential of some peptides, such as α-9 (57–68) and α-2 (62–75), was confirmed when they were recognised by a T cell line derived from Norwegian CD patients.43 Gliadin peptide 31–43 promotes an endoplasmic reticulum-stress pathway by inducing Ca2+ mobilisation from the endoplasmic reticulum, whereas peptides 31–43 and 57–68 can induce immune dysfunction.44 In addition, peptides 31–43 and 57–68 can alter immune regulators and induce deamidation of gluten peptides and gliadin–tissue transglutaminase crosslinking in enterocytes.36,43 As most of the peptides are immunogenic, some of them (p10-mer, QQPQDAVQPF) have protective effects that prevent gliadin-dependent dendritic cell maturation (Table 1).45
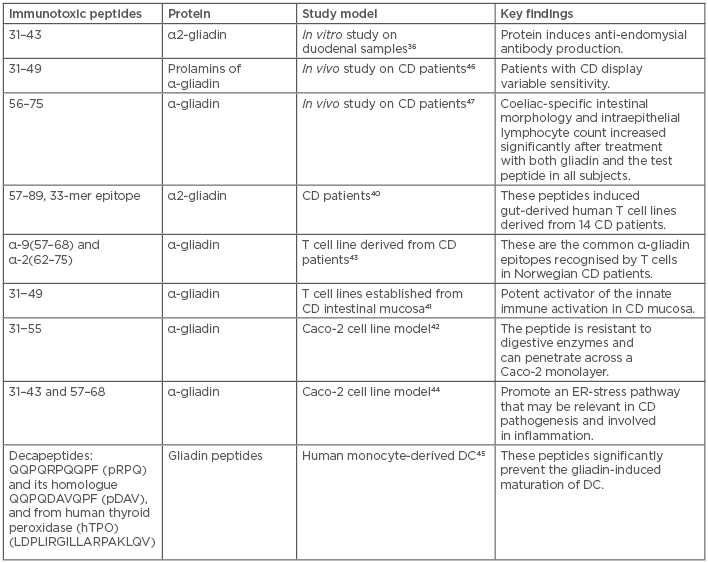
Table 1: Immunotoxic gluten peptides and their diverse effects.
CD: coeliac disease; DC: dendritic cell; ER: endoplasmic reticulum.
RNA INTERFERENCE AND WHEAT
A conserved biological response to double-stranded RNA, known as RNAi or post-transcriptional gene silencing, mediates resistance to both endogenous parasitic and exogenous pathogenic nucleic acids, and regulates the expression of protein-coding genes. RNAi has been cultivated as a means to manipulate gene expression experimentally and to probe gene function on a whole-genome scale.48 RNAi technology has been used to produce GM plants, and to provide benefits to CD patients. Gil-Humanes et al.49 designed hairpin constructs that are expressed in the endosperm of bread wheat and have the potential to downregulate gliadin proteins in the transgenic lines. Three transgenic wheat lines did not elicit T cell responses during in vitro treatment with T cell clones derived from intestinal lesions of CD patients. Another study by the same group found that the downregulation of γ-gliadins proved beneficial for enhancing the quality of the dough.50 The subsequent transgenic clones resulted in stronger dough quality that had tolerance to over-mixing, from an industry perspective. In a recent study,51 all of the gliadin fraction of wheat was successfully downregulated using RNAi so that the new wheat line exhibited stability and tolerance to over-mixing, thereby showing better bread-making qualities. Continuing their work on previous wheat lines, they evaluated the physical properties and contents of gliadins, thus further predicting the amount of safe bread intake possible for CD patients. Hence, Gil-Humanes et al.52 claimed their wheat line possessed similar baking and sensory properties to normal flour but lacked 97% of the gliadin content. The wheat they developed also had better nutritional properties because of higher content of lysine, an essential amino acid. Although, no clinical trial was performed in this study, a safe consumption of 67 g of their bread per day by the CD patients was claimed as per descriptions of per day maximum safe limits of gluten intake by Catassi et al.53
The ω5-gliadins are the major sensitising allergens in WDEIA, a disorder in which a patient experiences an allergic response during exercise. To contain such a response, Altenbach et al.54 generated transgenic lines of wheat that were knocked down for ω5-gliadins. Furthermore, in a set of additional experiments, it was revealed that the protein content of flour was determined by the fertiliser regime in both transgenic samples as well as normal wheat samples. Subsequently, ω5-gliadin was also indicated to have a negative effect on flour quality; thereby, suggesting that transgenic wheat lines produce better flour quality than wild-type wheat.
Altenbach et al.55 further observed that the allergenic response of ω5-gliadins knock-down wheat lines was reduced in patients, as determined by serum immunoglobulin E reactivity in a clinical trial. Two transgenic wheat lines were assessed for their allergenic potential, in which ω5-gliadin genes were silenced by RNAi. Sera from 7 of 11 WDEIA patients showed greatly reduced levels of immunoglobulin E reactivity to ω5-gliadins for both transgenic lines. However, the sera also showed low levels of reactivity to other gluten proteins, but sera from three patients showed the greatest reactivity to proteins other than ω5-gliadins, which included either high molecular weight glutenin subunits (HMW-GS), α-gliadins, or non-gluten proteins. The complexity of immunological responses among these patients suggests that flour from the transgenic lines would not be suitable for individuals diagnosed with WDEIA. To the best of the authors’ knowledge, transgenic wheat varieties knocked down for α-gliadins have not yet entered clinical trials for CD in humans.56 However, the present trial of ω5-gliadins revealed that its administration in the WDEIA population could reduce the incidence of this food allergy.55 The current clinical trial has also raised questions on the applicability of transgenic lines, thereby pointing towards the complexity of WAD; i.e., WDEIA, CD, and wheat allergy, and the complex status of overall wheat proteins.
As the wide range of complex immunogenic proteins of wheat is still a challenge in developing immune-tolerant wheat lines, wheat proteins as a whole should be targeted while trying to give an alternative to the GFD. This study also raised questions on the use of such silenced GM plants even after a number of quality checks. The effect of gene knockdown on other proteins and metabolites that remain untraceable by current molecular techniques is another challenge regarding GM plants. Furthermore, what makes other proteins immunogenic for the WDEIA population is still not evident, and whether it is because of a changed proportion of a targeted protein component, a consequence of the gene silencing on the plant’s metabolic control, or an imbalanced proportion of proteins is a subject of further investigations. Gil-Humanes et al.57 reported that silencing induced a strong reduction in all the gliadins but caused a compensatory effect on the synthesis of non-gluten proteins by upregulation.
Furthermore, the effect of gliadin knockdown on nutritional values of wheat remained contradictory; however, Barro et al.58 attempted to solve this by validating that silencing WAD-associated proteins did not affect the total protein and starch content of wheat. The authors designed a combination of seven plasmids containing RNAi fragments to mask all major components of WAD; i.e., α, γ, ω-gliadins, and low molecular weight glutenin subunits. Out of these combinations, two provided a >90% reduction in gluten content in comparison with the wild-type when measured by anti-gliadin 33-mer monoclonal antibody. However, total protein and starch contents remained unaffected in all types of combinations. Gel electrophoresis, reversed-phase high-performance liquid chromatography, and liquid chromatography–mass spectrometry were used to measure the extent of silencing. Though promising results were also observed in experiments by Altenbach et al.,54 questions will remain unanswered until preclinical and clinical trials likewise yield convincing results. Table 2 represents information about the different RNAi approaches used to produce safe wheat lines, and their characteristic features.
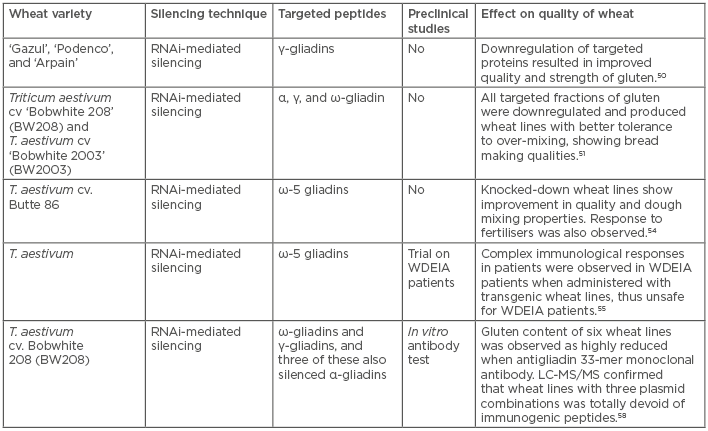
Table 2: RNA interference approaches to mask the expression of immunogenic proteins and their clinical relevance.
LC: liquid chromatography; MS: mass spectrometry; RNAi: RNA interference; WDEIA: wheat-dependent exercise-induced anaphylaxis.
FERTILISERS AS PREDOMINATING FACTORS FOR ACCUMULATION OF RISK PROTEINS IN WHEAT
Currently, both the prevalence and incidence of food intolerances are increasing,59-62 but the reason for widespread food intolerance is unknown. Studies have reported the adverse effects of sulfur fertilisers on gene regulation of wheat plants, thereby increasing the content of risk proteins (immunogenic proteins i.e., gliadins).63,64 These adverse effects due to genomic level changes need validations using metabolomics and other omics approaches integrated with clinical practice or trials in suitable models. Furthermore, the dysregulations at the genetic level that modulate the metabolic profiles of plants is a food safety concern.65,66
Altenbach et al.63 revealed that environmental factors, including fertilisers, affected the composition of specific flour proteins and their regulation. While conducting experiments, Triticum aestivum was grown with and without post-anthesis fertilisation (PAF), followed by quantitative two-dimensional gel electrophoresis of the flour. Subsequently, proteomic profiling clarified that the proportions of 54 unique proteins were altered in the treatment group; PAF treatment resulted in an increased proportion of most ω-gliadins, HMW-GS, serpins, and some α-gliadins.
Hurkman et al.,64 while conducting studies to observe the differential effects of temperature and fertiliser treatment on the development and yield of grain, reported that both the treatments elicited the accumulation of gluten proteins in wheat flour. Under high temperature conditions, gluten protein accumulation was not observed by PAF treatment, but the majority of HMW-GS, ω-gliadins, and some α-gliadins were found to be elevated, thereby affirming that environmental stimuli do influence the accumulation of risk proteins of CD. The studies presented provide evidence that the man-made means for increasing productivity of crops can be a prominent cause of food intolerances because of a lack of food safety measures. The aforementioned studies highlight the adverse effects of sulfur in terms of food safety and that they need to be validated by targeting studies to evaluate the effect of sulfur-induced metabolome change and its effect on food safety in relation to human health. Although there is lack of literature in this regard, there is some evidence in support of the side effects of sulfur fertiliser in GM wheat, as described in the next section.
CROSS TALK ON RNA INTERFERENCE, FOOD SAFETY, AND METABOLOMICS IN WHEAT
It is worthwhile to elucidate the unwarranted side effects of knocked-down wheat lines of α-gliadins on plant metabolites since α-gliadins contain sulfur-rich amino acids, including cysteine and methionine. Furthermore, in wheat lines knocked down for α-gliadin genes, sulfur amino acids used in the synthesis of α-gliadin might remain accumulated in the plant.67,68 The accumulation of sulfur is reported to induce its incorporation into other plant metabolites that may become a food safety concern, but this was not observed in metabolomic studies in transgenic versus wild lines.69,70 Therefore, with the support of metabolomics, the recent studies advocated that transgenic varieties are safe, because no change in the metabolomic signature in transgenic verses wild wheat lines was observed.
RNAi-produced wheat lines lack antigenic components but result in diverse side effects on the plants. Initially, evaluation was performed by proteomic analysis of different parts of the plant (grains, leaves, straw, and husk).39,40 Comparison of proteomic profiles of transgenic lines with that of normal lines gives an overview of the disturbed gene function or a description of knocked-down genes.63,64 RNAi-induced silencing of 75 α-gliadin genes that completely eliminated all gliadin proteins, as confirmed by proteome analysis,71 raises the safety issues70,72 related to such varieties; these should be considered a priority, especially those that are caused by a disturbance in a single plant metabolite or whole metabolome,73 with and without treatment with fertilisers. Recently, metabolomics has helped in better understanding the effects of any external stimuli (fertiliser) or genetic modifications (gene knockdown) on plant metabolism and the associated issues of biosafety.
Zörb et al.69 performed gas chromatography mass spectrometry-based metabolite profiling of flour of wheat line silenced for 75 α-gliadin genes and reported that, in comparison to wild-type plants, no appreciable difference in 109 metabolites was seen when plants were grown without sulfur. No unintended side effects of RNAi-induced gene silencing were observed. Conversely, the effect of fertilisation or single nutrient (sulfur) availability in disturbing metabolomic status was much higher than RNAi-induced silencing. The concentration of metabolites was also found to increase with increasing sulfur supply. Zörb et al.69 also revealed that variable amounts of sulfur supply influenced the yield aspects of grain metabolome in both the wild-type and transgenic wheat line. Plants grown with higher sulfur content showed elevated grain metabolite concentration in comparison to the plants grown without sulfur treatment. Moreover, principal component analysis showed that the levels of β-amino isobutyric acid were affected the most by variation in sulfur supply in wild-type wheat. Furthermore, when α-gliadins were knocked down, alanine, glycine, serine, homoserine, and tyrosine were found to be associated with sulfur-induced differences in the grains. These studies confirm the effects of sulfur fertilisers in GM wheat but, noting the concerns discussed above it is pertinent to elucidate the effects of sulfur alone on gene regulation and also the associated metabolomics in order to make the food safety concerns relevant.
Collectively, it can be concluded that sulfur was not only potent in inducing genetic dysregulations in wheat,63 but it might have produced metabolic changes that were reflected in metabolomics studies.69
CONCLUSION
The results of various experiments related to proteomics and metabolomics are in accordance with each other and reveal that fertilisers affect the proteome and metabolome of wheat flour; thereby, raising issues related to food safety. Although RNAi-induced gene silencing does not affect the metabolomic signature of wheat flour, fertilisers do have an effect. Sulfur fertilisers remain the determining factor for disturbance in plant metabolism, followed by dysregulation in the proteome. Moreover, proteomic studies demonstrate that sulfur fertilisers induce the accumulation of gliadins in wheat, which makes the flour more immunogenic. Thus, the techniques adopted are useful for validating that fertilisers induce metabolic disturbances in plants. However, clinical trials of wheat lines knocked down for ω-gliadin showed reduction in disease-specific markers in WDEIA patients, but reactivity to other wheat protein components could not be ruled out. This raises controversy on the gene expression status of other gluten proteins that may be dysregulated as a consequence of interference exerted by RNAi. Therefore, though omics techniques are useful to validate the global metabolic control or gene expression status, initial in vitro or in vivo preclinical studies are needed to guide better future clinical output. Future research in this field should be attributed to validation of food safety issues through improved platforms of studying the transcriptome, proteome, metabolome, and immunome.