Abstract
In this editorial, the authors highlight newly licensed medications for the symptomatic treatment of Parkinson’s disease (PD) and their role in the current management of PD. Non-motor symptoms (NMS), which cause significant disability in this patient group, are highlighted to emphasize their importance as targets for treatment, both in current and in future research. The clinical trial pipeline for disease-modifying treatments for PD is discussed with an emphasis on therapies targeting α-synuclein propagation and aggregation within the central nervous system. Newly licensed treatments and advances in the management of motor complications are discussed. Finally, the current state of clinical trial design is highlighted, with a focus on how study design is evolving and the potential implications for both the treatment and prevention of PD.
Key Points
1. Parkinson’s disease (PD) is the fastest rising cause of neurological disability and death worldwide. Over the last decade, significant advancements in understanding the clinical spectrum and biological underpinnings of PD have led to the introduction of new medications and therapies.
2. This article provides a comprehensive overview of new infusion therapies, sublingual and inhaled formulations of established compounds, and the evolution of deep brain stimulation (DBS) capabilities.
3. Health care professionals should understand the significant impact of non-motor symptoms (NMS) on patients’ clinical course. They should also be aware of the marked expansion of clinical drug and device trials for PD, highlighting the ongoing efforts to develop disease-modifying therapies.
INTRODUCTION
Parkinson’s disease (PD) is a progressive neurodegenerative disorder. Current diagnostic criteria rely predominantly on clinical features, specifically the presence of motor symptoms, including bradykinesia, in combination with extrapyramidal rigidity and/or resting tremor.1 Of all the neurological disorders, PD is now recognized to be the fastest rising cause of disability and death worldwide.2 Over the last decade, there have been dramatic steps in our understanding of both the clinical spectrum and biological underpinnings of the disease. These developments have led to the introduction of new medications into clinical practice, including novel infusion therapies and both sublingual and inhaled formulations of established compounds. There has also been an ongoing evolution of the capabilities of established therapies, including deep brain stimulation (DBS), which is generally used for individuals with advanced PD, but is potentially beneficial also in early-stage PD.3 This has paralleled a significant expansion in the number of clinical drug and device trials for PD. As of January 31, 2024, there were 136 active trials investigating medications for PD. A total of 44% was considered as disease-modifying therapies and the remaining 56% was symptomatic therapies. McFarthing et al.4 have described these trials in detail.
THE CLINICAL SPECTRUM OF PARKINSON’S DISEASE
“Early” Parkinson’s Disease
The motor symptoms of early PD are often the clinician’s focus over the first few years following diagnosis, which in most cases remains based on the detection of clinical symptoms. In this regard, the Movement Disorder Society (MDS) has provided clinical diagnostic criteria for PD that are intended for use in clinical research and for guiding clinical diagnosis.1 First-line symptomatic therapies for motor symptoms remain unchanged over the last decade and include levodopa preparations, dopamine agonists, and monoamine oxidase inhibitors (MAOi). The evidence base and rationale for selecting between medications is summarized in the evidence-based medicine review commissioned by the MDS.5 Non-motor symptoms (NMS), including neuropsychiatric, neurocognitive, sleep, and autonomic impairment, are now also recognized to have a significant impact on a patient’s clinical course.6,7 It has recently been observed that, in patients with early PD, the presence of depression may be as significant a predictor of impairment in activities of daily living as motor function (Stewart et al., unpublished data). Depression is significantly associated with the development of cognitive decline in PD,8 and there is observational evidence that early treatment of depression and anxiety may improve both non-motor and motor outcomes.7 Hence, for clinicians, it is important to remember that NMSs should be actively explored, rather than focusing solely on easily visible motor symptoms.
“Advanced” Parkinson’s Disease
As the disease progresses, many of the early motor and NMSs worsen. A diverse range of symptoms, including gait impairment, postural instability, freezing, and neuropsychiatric and neurocognitive impairment, are more likely to occur and progressively worsen in the later stages of the disease.6 Where patients initially have a good clinical response to dopaminergic therapy, typically, the severity of motor symptoms and the probability of developing motor complications increase as time passes.9
Motor complications encompass OFF periods, which are specific times during the day when dopaminergic medications exhibit reduced efficacy; OFF dystonia, which typically presents painful posturing of a limb during an OFF period; and dyskinesias, characterized by involuntary hyperkinetic movements affecting any part of the body. These can all greatly affect a person’s quality of life. In clinic, first-line management strategies for motor complications include increasingly complex medication regimens. Clinicians and their patients can select one or a combination of different strategies, including increasing the dosing intervals of standard-release dopaminergic medications, the addition of dopamine agonists, prolonged-release levodopa formulations, or enzyme inhibitors, including MAOis and catechol-O-methyl transferase inhibitors (COMTi). Although it is not available in Europe, in 2019, the FDA approved a drug with a new mechanism of action, istradefylline (a selective A2A receptor antagonist), for the treatment of motor complications. A pooled analysis of all studies showed a reduction in OFF time of 0.45 hours compared to placebo (p<0.0001).10
Whereas enzyme inhibitors prolong the ON time and minimize the OFF time over a 24-hour period, over the last few years, several drugs have been released, which provide ‘on-demand’ relief for the same symptoms. In 2018, the FDA approved Inbrija® (Merz, Frankfurt, Germany), an inhaled levodopa formulation. When taken at a single dose, an individual’s PD motor symptoms begin to show a significant improvement compared to placebo at 10 minutes post-dose.11 The FDA approved a further ‘on-demand’ therapy, sublingual apomorphine, in 2020. Again, significantly more patients demonstrated improvement in their PD motor scores compared to placebo at 15 minutes.12 Both therapies, which are modified formulations of established drugs, enhance outcomes notably in patients with unreliable gastrointestinal absorption of oral medications, a frequent symptom in individuals with PD.
If first-line oral and ‘on-demand’ medications fail to sufficiently improve an individual’s quality of life, then device-aided therapies (DAT) are often considered. These therapies aim to minimize the fluctuation index of dopaminergic preparations by either instilling them as a continuous infusion or bypassing peripheral dopaminergic pathways and applying high- frequency stimulation directly to targets within the basal ganglia network, which have been identified as playing a key role in mediating the motor symptoms of PD, i.e., DBS.
Prodromal Disease
It is well established that the neurodegenerative process in PD begins decades before the onset of clinical symptoms (preclinical phase), and later, symptoms and signs may be present but be insufficient to define the disease (prodromal phase). In fact, patients with PD have frequently experienced one or multiple NMSs for years prior to their diagnosis. Autonomic dysfunction, including constipation and urinary urgency, as well as rapid eye movement sleep behavioural disorder (RBD), depression, anxiety, and hyposmia are among the most recognized prodromal disorders. The annual risk of developing a synucleinopathy, such as PD, dementia with Lewy bodies, or multiple system atrophy, for someone with RBD is 6–7%. After 12 years of follow-up, 73.5% of patients will have converted.13
There is an increasing emphasis on developing tests to aid researchers and clinicians in predicting which patients may be at increased risk of phenoconverting soon, potentially providing a further avenue for intervention before the onset of motor symptoms. The MDS has released the research criteria for prodromal PD, which provide an evidence-based methodological framework to statistically estimate the likelihood that an individual has prodromal PD.14 The utility of these criteria in clinical settings, however, remains to be established.
The Parkinson Associated Risk Syndrome (PARS) study found that a positive cerebrospinal fluid (CSF) α-synuclein seed amplification assay (SAA) was detected in 48% of individuals with hyposmia, compared to 4% of normosmics. Individuals with hyposmia who tested positive for SAA had an increased relative risk (RR) of reduced dopamine transporter activity, as identified by a dopamine transporter scan (RR: 3.26; 95% CI: 1.16–9.16). Additionally, seven out of 12 SAA-positive individuals with hyposmia and a dopamine transporter deficit subsequently developed symptoms consistent with synucleinopathy.15 This and other supporting data suggest that biomarkers such as SAA positivity and dopamine transporter deficit may precede the onset of clinical synucleinopathy.15 Stratifying individuals with symptoms more common in the years prior to PD, depending on these biomarkers, provides a potential mechanism wherein their chance of developing diagnostic motor or cognitive symptoms may be predicted in the future. The Parkinson’s Progression Marker Initiative (PPMI), a large multicenter observational study, has recently embarked on a large effort to recruit individuals with hyposmia and RBD to advance research in this area.16
THE BIOLOGICAL UNDERSTANDING OF PARKINSON’S DISEASE
The Role of α-Synuclein
PD is a heterogeneous condition, both in terms of its clinical picture and of its underlying biological mechanisms. Histopathological examinations reveal that most patients exhibit the characteristic findings of neuronal Lewy bodies. These are abnormal protein deposits composed of multiple different proteins, including abnormally folded α-synuclein. A leading hypothesis in the field is that misfolded α-synuclein monomers exhibit prion-like activity. They propagate throughout the nervous system, leading to subsequent characteristic but variable patterns of neuronal damage and subsequent clinical symptoms that reflect the topographical burden of Lewy body deposition. For a recent update on the scientific background of this hypothesis, refer to Jan et al.17
Many clinical trials are either ongoing or have recently been completed, wherein interrupting the spread of α-synuclein or enhancing its clearance is the main goal of treatment. However, no trial has yet met its primary outcome. Post-hoc analyses have provided some hope that a potential disease-modifying therapy may be anticipated. The PASADENA study investigated whether prasinezumab, a monoclonal antibody that binds to aggregated α-synuclein, thereby potentially enhancing its clearance, slowed disease progression in early PD.18 While there was no significant difference compared to placebo over 1 year in the primary outcome, minimal progression was observed in motor outcomes in both the treated and untreated groups. Overall, there was a lower level of motor progression in the treatment group. In the open label extension study, patients on active treatment had better motor outcomes over 4 years when compared to controls from an external comparator natural history study, the PPMI study.19 Post-hoc analyses of the PASADENA study also suggested that patients in a prespecified subgroup with faster motor progression had better motor outcomes on treatment.20 Consequently, a larger and longer Phase III study has recently completed and is due to read out shortly. As of January 2024, there are nine clinical trials underway using different molecules that directly target α-synuclein.4
In 2019 and 2021, two studies were published that validated a highly sensitive and specific diagnostic test for PD: the CSF α-synuclein SAA.21,22 The test is positive in 95% of post-mortem confirmed PD.23 The pooled sensitivity and specificity of the test to differentiate synucleinopathies from controls were 0.88 and 0.95, respectively.24 In addition to providing an extra diagnostic tool, a reliable biomarker also means that clinical trials can be enriched, i.e., patients who are α-synuclein-negative need not be enrolled in studies of compounds targeting the α-synuclein pathway. It has also led to two proposals for a biological definition of PD, and one of these groups has proposed a subsequent staging system, akin to that observed in Alzheimer’s disease.25-27 The staging system proposes the use of the term “neuronal α-synuclein disease,” taking into account that patients with prodromal disorders may have positive SAA assays and are at increased risk of developing PD, compared to SAA-negative individuals.28,29 These proposals are currently intended as research criteria, as SAA testing and, to a lesser extent, genetic evaluation are not widely available in clinical practice. The concept, however, is particularly exciting as it has led academic and industry partners to consider disease-modifying treatment trials in patients with prodromal disease, with the aim of preventing the development of PD in these patients (see section Approaches to Clinical Trials in Parkinson’s Disease).
Other Molecular Mechanisms Underlying Parkinson’s Disease
There remain, however, several unanswered questions. The most intriguing question for the authors is what are the upstream mechanisms that have triggered the initial seeding of abnormal α-synuclein monomers and what is the mechanism behind the development of PD in patients who are both SAA-negative and have no evidence of Lewy body disease at post-mortem. Indeed, while α-synuclein is widely considered an important pathology and therapeutic target, several researchers have proposed that the field may be overly focused on the pathogenic role of α-synuclein in PD, with important implications for a large number of anti-synuclein therapies in development.30,31 Genetic differences in patients with PD may provide important clues. Variants in the GBA and LRRK2 genes are the most common cause of autosomal dominant PD, while mutations in the PRKN gene are the most common cause of autosomal recessive PD.32 In one large analysis, only 67.5% of patients with LRRK2 PD were SAA-positive29 compared to 93.3% of patients with sporadic PD and 95.9% of patients with GBA PD. The CSF SAA has not been used in a large population of individuals with PRKN PD, but previous post-mortem studies have suggested that more than 50% of PRKN PD do not have Lewy body pathology.33
Anti-synuclein therapies are unlikely to have a significant impact on SAA-negative PD. It remains unclear whether these individuals require an entirely different approach to disease-modifying therapies. Initiatives such as the LRRK2 Initiative Therapeutics Exchange (LITE)34 seek to explore new biomarkers for individuals who carry the LRRK2 variant and are more likely to be SAA-negative. In the interim, there are also a number of drugs in therapeutic trials that do not directly target the removal of α-synuclein. Currently, there are nine compounds targeting LRRK2 and GBA, which are encoded by the LRRK2 and GBA genes, respectively, undergoing clinical trials. The studies are divided between those targeting discrete subpopulations of individuals with confirmed genetic variants and those that have incorporated individuals with PD, unaware of their underlying genetic background on the basis that the activity of these enzymes is also abnormal in sporadic PD.
In addition to the aforementioned drug classes, current disease-modifying trials target other mechanisms, such as neuroinflammation suppression, antioxidant activity, gut microbiome manipulation, neuronal survival, and lysosomal and mitochondrial function. A full review is beyond the scope of this editorial but readers are recommended to read the recent summary by Vijiaratnam et al.35
DEVICE-AIDED THERAPIES
Infusion Therapies
Data from large cohorts of individuals with PD show that motor fluctuations (characterized by the development of OFF periods) occur in 54.3% and 100% of patients at 5 and 10 years, respectively. Levodopa-induced dyskinesias occur in 14.5% and 55.7% over the same period (Figure 1).36 While a significant number of patients can be managed with adjustments to oral medications, many will develop motor complications that are unresponsive to these treatments. At this stage, provided there are no clear contraindications, there is an expanding array of DAT available, which can effectively address dopamine-responsive symptoms. It is important to note that symptoms which do not respond to oral dopaminergic therapies, such as cognition issues, balance problems, and ON freezing, will also not respond to continuous dopamine delivery via DAT.
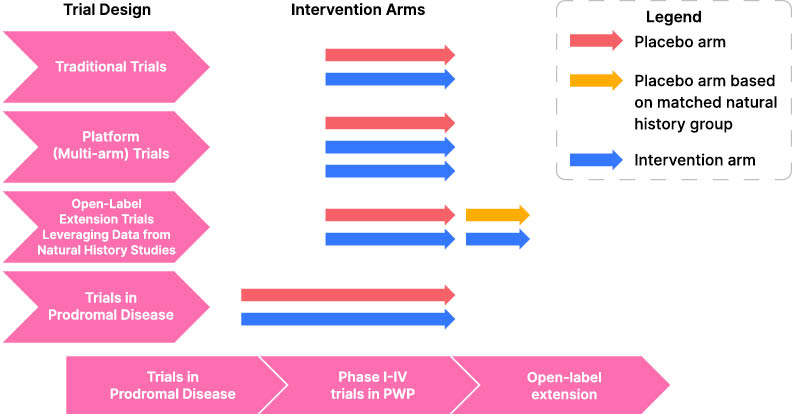
Figure 1: An outline of novel trial designs for testing disease modifying treatments in Parkinson’s disease.
EEG: electroencephalogram; GCS: grapheme-color synesthesia; F: female; M: male.
This underscores the necessity of conducting a clinician-observed levodopa challenge test as part of the evaluation process for all DAT candidates.
DAT can be divided into continuous infusions and DBS. Apomorphine is a D2, D3, and D5 receptor dopamine agonist. It is currently available as cartridge for subcutaneous injection and as a sublingual film in the USA, but in Europe it is also widely available as a continuous subcutaneous infusion. As an infusion, it has been shown to reduce OFF time by a mean of 1.89 hours when compared to placebo (p=0.0025).37 Continuous subcutaneous infusions for PD have gained significant physician and public interest over the last year with the introduction of foslevodopa–foscarbidopa, a prodrug of levodopa. Similar to apomorphine, trial data have demonstrated that it can reduce OFF time by a mean of 1.79 hours (p=0.0054) compared to placebo.38 Levodopa can also be administered through infusion directly into the small intestine via a percutaneous endoscopic gastrostomy with jejunal extension (PEG-J). Carbidopa/levodopa (Duopa/Duodopa®, AbbVie Inc., Chicago, Illinois, USA) was first licensed in 2015 and it is effective at improving motor complications (OFF time was reduced by a mean of 1.91 hours compared to placebo; p=0.0015 in the pivotal Phase III study).39 In 2019, Sweden approved an alternative intestinal infusion that combines levodopa, carbidopa, and entacapone, which has gained wider use in Europe but, is yet to pass FDA regulatory approval. The addition of entacapone, a COMTi, allows for lower doses of levodopa, meaning the pumps are smaller and potentially reducing levodopa-related side effects, such as peripheral neuropathy, although there have been no head-to-head studies.40
Deep Brain Stimulation
DBS of either the subthalamic nucleus (STN) or globus pallidus interna is the primary neurosurgical alternative to infusion therapies for refractory motor complications. The original studies and a subsequent meta-analysis highlighted that STN DBS reduces OFF symptoms by an average of 60%. Dyskinesias are reduced by 60–80%, in part, due to an average of 30–50% reduction in the dose of oral dopaminergic medications.41–43
DBS has the added advantage over other forms of DAT in providing a significant degree of tremor control beyond what dopamine-based treatments can provide, through the stimulation of non-dopaminergic tremor networks. This makes it first-line when considering DAT for individuals with significant tremor.
DBS has experienced numerous substantial advancements in recent years. Improvements in battery life and electrode design have meant that patients need to recharge less frequently and clinicians can compensate for electrode misplacement using multisegmented electrodes. These allow steering of current toward target structures, thereby improving clinical outcomes, minimizing side effects, and reducing the need for repeat operations to move mildly misplaced electrodes. While not available across all devices, one company has developed the ability to perform remote (out of clinic) programming, reducing the number of inpatient clinic visits for patients.44 Other manufacturers have focused on the ability to incorporate anatomical imaging into programmers, allowing clinicians to target the “sweet spot” areas of stimulation using multicontact electrodes. This reduces the time needed for traditional trial–and-error programming, wherein each contact along electrodes containing 4–16 different points of stimulation needs to be tested for both efficacy and side effect thresholds, before selecting the optimal contact.
In 2021, the FDA approved a novel device the Percept™ PC (Medtronic, Minneapolis, Minnestoa, USA), which has the unique ability among currently available commercial DBS models of being able to detect electrical activity as well as deliver stimulation. The electrodes record local field potentials (LFP). LFPs are created by synchronous activity in interconnected populations of neurons, occurring due to the synchronizing effects of emergent network oscillations.45 A specific signal in the low beta frequency is a reliable and reproducible correlate of clinically detectable bradykinesia and rigidity.46 It is supressed by both dopaminergic medications and stimulation.47,48 The band can be tracked, allowing clinicians to focus stimulation on contacts recording the highest beta peak and target the degree of stimulation to the peak in real-time. As the stimulation increases, the spectral power of the peak reduces, with concomitant improvement in motor symptoms. This has resulted in a new way of programming that does not rely on trial-and-error approaches or anatomical review. The ability to track LFPs in real-time means that patients can also log when they experience specific symptoms. The LFP data can be interrogated in clinic, and stimulation parameters or medications can be subsequently adjusted to improve clinical outcomes.
The Adapt-PD trial has taken this a step further and used LFP data to vary stimulation parameters in real time, increasing and decreasing stimulation amplitude in response to variation in beta peak power.50 The study has not been published yet, but early presentations at international conferences have suggested that using “adaptive-DBS” improved patient “ON” time by up to 1.4±3 hours compared to continuous DBS (p<0.0125).49,50 This may lead to a system that can vary treatment in a much more physiological manner than any other symptomatic treatment so far. Although oral therapies lead to pulsatile pharmacokinetics of levodopa and infusion therapies result in continuous levodopa pharmacokinetics, it has become increasingly recognized from LFP data that the network oscillations that reflect the akinetic-rigid symptoms of PD are neither fixed-interval pulsatile nor continuous and constant. They vary depending on diurnal rhythms, physical activity, and emotional state.51 This should be evident from the fact individuals with PD still experience OFF time and dyskinesias, albeit to a lesser degree, even on continuous infusion therapies. One small study effectively demonstrated that LFP activity can be influenced by emotional state. They documented that the beta frequency band was suppressed over Christmas in four patients in the absence of any medication changes, demonstrating how LFP activity, and by inference bradykinesia and rigidity, can improve over festive periods.51,52 A system that can vary treatment of network oscillations in a more physiological manner has the potential to provide better control of motor complications (Figure 2).
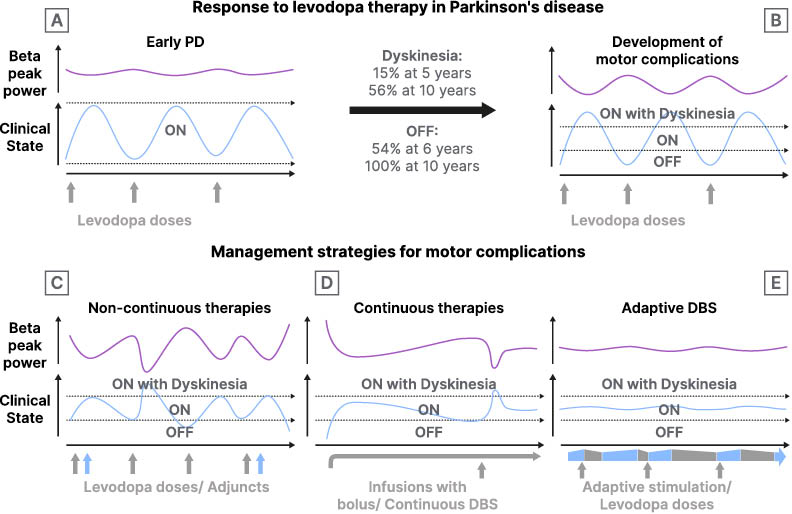
Figure 2: A pictograph demonstrating the development of motor complications in Parkinson’s disease and the current and potential future management strategies.
A) In early PD clinical symptoms and local field potential activity (beta peak power) are controlled with doses of oral levodopa (grey vertical arrows). B) When motor complications develop, the therapeutic window narrows, individuals with PD experience OFF periods and dyskinesia. Clinical symptoms correlate with a fluctuation in beta peak power. C) The doses of levodopa can be fractionated, and adjuncts such as monoamine oxidase inhibitors (MAOi) and catechol-O-methyl transferase inhibitors (COMTi) added (blue vertical arrows), to improve time within the therapeutic window. D) Continuous infusions increase time within the therapeutic window. Fluctuations in clinical symptoms and concomitant beta peak power still occur but are managed by bolus doses of the same infusion. E) Potential role of adaptive DBS, stimulation is varied in response to beta peak power in real-time allowing adjustment of stimulation amplitude over seconds, thereby minimizing motor complications. Oral dopaminergic medications are still required but at lower doses (grey vertical arrows). Adaptive DBS will vary over the course of seconds to minutes, rather than hours as shown in the figure. The slower time course was used because it is more suitable for the visual representation.
DBS: deep brain stimulation; PD: Parkinson’s disease.
NOVEL TREATMENTS FOR PARKINSONIAN TREMOR
There is increasing recognition that, as well as being a key diagnostic marker for PD, parkinsonian tremor is one of the most troublesome symptoms for many. Pasquini et al.53 highlighted that 96% of individuals with PD will experience tremor in the first 7 years of their condition. While classically this tremor may be considered predominantly a rest tremor, 85% of individuals will have a postural tremor and 79% will have a kinetic tremor.53 Recent patient survey data have revealed that 49% of individuals with PD feel that there is either no or minimal improvement in their tremor from current medications.54 Postural and kinetic tremors lead to higher functional impairment and are less likely to respond to medications.53 As discussed, DBS is an effective treatment for tremor; however, there is a group of individuals who may be unsuitable for invasive surgery, develop significant tremor at an advanced age, or be concerned about the risks inherent to a neurosurgical procedure. There are various pharmacological and non-pharmacological strategies currently in development. The authors would like to highlight two potential strategies that have gained recent interest: targeted botulinum toxin injections and MRI-guided focused ultrasound.
Targeted Botulinum Toxin Injections
A double-blind, placebo-controlled crossover trial demonstrated that injections of botulinum toxin into arm muscles can improve clinical rating scores for rest, action, and postural tremor, alongside patient perception of improvement. In experienced hands, there were minimal side effects.55,56 Patient outcomes may be improved by using electromyography guidance, i.e., the “Yale technique” or sensor-based kinematics to aid selection of the appropriate muscles at effective doses.
MRI-Guided Focused Ultrasound
MRI-guided focused ultrasound (MRgFUS) is now increasingly available for the treatment of essential tremor (ET), and there is renewed interest in its potential role in PD. A randomized trial of unilateral MRgFUS thalamotomy (targeting the ventralis intermediate nucleus [Vim], a target typically considered in the management of ET) for individuals with tremor-dominant PD demonstrated that patients’ tremor scores improved by a median of 62%.57 Ablation of the Vim is expected to affect tremor significantly but it may not substantially improve bradykinesia or rigidity. Unilateral ablation of the subthalamic nucleus (STN; the same target as that often used in DBS for PD) has also been explored in open label studies and appears to have potential for providing a meaningful and sustained improvement in contralateral motor function.58 While early data suggest that the clinical improvement from MRgFUS thalamotomy (Vim) may not be sustained to the same degree as in ET, and the therapy is currently limited to unilateral or staged bilateral use, the therapy remains a potential option for surgically unsuitable patients with disabling tremor. Further research is needed before this can be considered an established therapy.
APPROACH TO CLINICAL TRIALS IN PARKINSON’S DISEASE
Alongside the increase in drug trials for PD, there is a growing recognition that current research trial design is cumbersome and expensive. An individual study drug takes, on average, 7.3 years to progress from Phase I studies to subsequent market authorization.59 Several initiatives have been proposed to make the process more economical in terms of both time and cost. The PD multi-arm platform study60 is in late stage setup, with the goal of mimicking the success of the prostate cancer platform study “STAMPEDE”61 in creating a “‘platform” for a continuous study design with multiple trial arms so that multiple agents can be explored in parallel. There are also initiatives by longitudinal natural history studies, such as PPMI, to incorporate treatment trials into their study protocol, thereby providing the advantage of already having a substantial number of healthy controls and patients at different stages of PD enrolled, cutting down recruitment and investigation costs. The natural history of the disease from well-phenotyped cohorts can also be used as a surrogate (with caveats) for a placebo treatment arm in open-label extension studies, providing the pharmaceutical industry and the scientific community added confidence that longer studies for a specific agent may be worthwhile.19 The availability of SAA assays to identify CSF α-synuclein in prodromal PD has led to the recruitment of individuals with prodromal disorders, such as hyposmia and RBD, into large natural history studies such as PPMI. This offers a potential mechanism whereby trials of PD prevention can also be considered (Figure 1).62, 32 Finally, there is, in general, a lack of clinical trial data in non-Caucasian populations or low-income countries and this remains a very important issue to address in future clinical trials.
CONCLUSION
There have been many advances in treatments for PD over the last 5 years, which have allowed for a finer, personalized approach to symptomatic management of symptoms. On demand, dopaminergic medications can now be delivered via oral, nasal, subcutaneous, and sublingual routes. There are four different forms of continuous infusion available via subcutaneous or intra-jejunal route, all offering “on demand” bolus medications. In addition, advances in DBS devices have led to new methods of modulating the abnormal network oscillations that are now recognized to be a key biomarker of bradykinesia. While there are still no commercially available disease-modifying therapies, the presence of a new CSF biomarker of PD, α-synuclein, has allowed for more accurate diagnosis of both PD and prodromal disease. Innovations in the way that the drug trials are conducted have the potential to increase the efficiency, whereby new medications can be investigated and may be well utilized to explore a new focus of treatment, preventing or at least prolonging the time to onset of PD.