Meeting Summary
Advances in treatment offerings are moving beyond classical considerations around glucose control to focus on other aspects of the disease. Such advances include the development of treatments that address the high cardiovascular (CV) risk in patients with diabetes, or have novel mechanisms of action, and new technologies that will facilitate the future integration of care.
The proprotein convertase subtilisin-kexin type 9 (PCSK9) inhibitors represent a new therapeutic approach for managing CV risk in patients with diabetes. New clinical data recently generated from dedicated diabetes studies have established PCSK9 inhibitors as an efficacious and well-tolerated treatment option for patients with diabetes and persistently elevated low-density lipoprotein-cholesterol levels, despite optimised lipid-lowering therapy.
Treatments with novel mechanisms of action are also being investigated. Sotagliflozin, a dual inhibitor of the sodium-glucose cotransporter 1 (SGLT1) and sodium-glucose cotransporter 2 (SGLT2), may offer additional clinical benefits beyond those of existing selective SGLT2 inhibitors in patients with Type 1 diabetes mellitus. The sotagliflozin inTandem Phase III programme will provide valuable insights regarding the potential role of dual SGLT1 and SGLT2 inhibitors as an adjunct to insulin therapy in patients with Type 1 diabetes mellitus.
Furthermore, advances in diabetes devices, such as implantable drug delivery systems, non-invasive glucose monitoring, and closed-loop artificial pancreas systems, are fuelling the development of new models of patient care. While there will inevitably be other innovations, three major advances will dramatically change diabetes care over the next 10–20 years: 1) digital diabetes health technologies, 2) artificial intelligence and machine learning, and 3) virtual reality.
Symposium Overview
Doctor Elizabeth Seaquist
Diabetes care is evolving. Advances in our understanding of diabetes pathophysiology and treatment now permit individualised therapy based on patient-centred treatment plans that provide the best evidence-based therapies, while minimising personal burden. This personalised approach to treatment requires that therapeutic goals go beyond glycated haemoglobin control to include patient identified outcomes of value such as side effects, cost, and minimal interference with daily living
Introduction
Professor Thomas Danne
HbA1c, a useful measure of glycaemic control, represents only a snapshot of a complex dynamic process.1 Mean HbA1c values cannot provide an accurate overview of day-to-day glycaemic variability,1 an increasingly important consideration in the management of diabetes and the development of new treatments that minimise the risk of hypoglycaemia. Accordingly, diabetes therapy offerings are evolving. Recent advances include treatments that target the elevated CV risk in patients with diabetes, treatments with novel mechanisms of actions, and new diabetes-related technologies that will be instrumental in revolutionising diabetes management. Such developments include the availability of PCSK9 inhibitors for managing hypercholesterolaemia in diabetes,2,3 dual inhibition of SGLT1 and SGLT2 as a novel therapeutic target for Type 1 diabetes mellitus,4 and new devices for improving care, e.g., implantable drug delivery systems, non-invasive glucose monitoring, and closed-loop artificial pancreas systems.
Proprotein Convertase Subtilisin-Kexin Type 9 Inhibition in the Treatment of Hypercholesterolaemia in Diabetes
Professor Dirk Müller-Wieland
Key Points
- Long-term exposure to elevated levels of low-density lipoprotein (LDL) particles is ‘toxic’ for the arterial wall; reducing LDL-cholesterol (LDL-C) levels should be a key focus of CV disease prevention.
- Individual CV risk represents one determinant of the therapeutic approach adopted for the reduction of LDL-C.
- Preliminary evidence generated from analyses of randomised clinical studies indicate that PCSK9 inhibitors effectively lower atherogenic lipoproteins and are associated with an acceptable safety profile, in both patients with and without diabetes.
An important challenge in the management of patients with diabetes is CV risk. In all individuals, lifelong exposure to raised concentrations of LDL-C increases the risk of experiencing a CV event.5 This risk is further elevated in patients with diabetes, irrespective of sex or age.6 Type 2 diabetes mellitus is associated with complex lipid profile aberrations, including elevated levels of very LDL, elevated numbers of LDL particles, and decreased levels of high-density lipoprotein cholesterol (HDL-C). While reduction of LDL-C is a primary goal in CV prevention, an increasing number of clinical guidelines and consensus statements are also incorporating recommendations for non-HDL-C treatment targets.7,8 Non-HDL-C, which accounts for all atherogenic lipoproteins, may represent a more appropriate predictor of CV risk than LDL-C alone for patients with diabetes and dyslipidaemia.7,8 Therapeutic approaches for LDL-C lowering in patients with diabetes and atherosclerotic CV disease (ASCVD) vary between clinical guidelines depending on risk categorisation (e.g., very high-risk versus extreme).8-10 The joint European Society of Cardiology (ESC) and European Atherosclerosis Society (EAS) 2017 guidelines recommend an LDL-C goal of <1.8 mmol/L (70 mg/dL) for patients with very high CV risk, while the joint American Association of Clinical Endocrinologists (AACE) and American College of Endocrinology (ACE) 2017 guidelines recommended a goal of <1.4 mmol/L (55 mg/dL) for patients with extreme CV risk.8,9 The American Diabetes Association (ADA) recommends consideration of the addition of ezetimibe to a moderate-intensity statin if a patient’s LDL-C is ≥1.3 mmol/L (50 mg/dL),10 guidance which is based on evidence from the IMPROVE-IT.11 The study demonstrated that in patients with a recent acute coronary syndrome, the combined simvastatin/ezetimibe treatment significantly reduced CV risk compared with simvastatin alone (median time-weighted average LDL-C levels: 1.4 mmol/L [53.7 mg/dL] versus 1.8 mmol/L [69.5 mg/dL], respectively; p<0.001).11 Furthermore, event rates for the primary endpoint at 7 years were significantly lower in patients with diabetes compared with those without diabetes (40.0% versus 45.5%; hazard ratio [HR]; 0.856; 95% confidence interval [CI]: 0.779–0.939; p=0.023).11 The relationship between intensive LDL-C lowering and reduced CV risk is well documented. Furthermore, randomised clinical studies have established a correlation between reduced LDL-C levels and regression of atherosclerotic plaque volume.12 A growing body of clinical evidence suggests that coronary atherosclerosis progression can be slowed by combining statins with additional LDL-C lowering agents. In a placebo-controlled, 78-week randomised study, combined therapy with a PCSK9 inhibitor and statin was associated with significantly greater reductions in absolute LDL-C and atherosclerotic plaque volume versus placebo (p<0.001).13 Importantly, post-hoc analyses demonstrated that the changes in plaque volume predominantly occurred at LDL-C levels >1.8 mmol/L (70 mg/dL).13
Proprotein Convertase Subtilisin-Kexin Type 9 Inhibition for Managing Low-Density Lipoprotein-Cholesterol Levels in Patients with Type 2 Diabetes Mellitus
In patients with Type 2 diabetes mellitus, targeting the LDL-receptor via PCSK9 inhibition represents an innovative approach for the management of LDL-C levels and CV risk.14 Preliminary insights from meta-analyses and pooled analyses of randomised clinical studies indicate that the PCSK9 inhibitors, evolocumab and alirocumab, markedly reduce atherogenic lipid levels in patients with diabetes, an effect that is consistent with observations in patients without diabetes.15,16 Furthermore, both evolocumab and alirocumab demonstrated safety and tolerability profiles that were comparable to placebo or active comparators and consistent with those observed in patients without diabetes.15,16 The effect of lowering LDL-C levels via PCSK9 inhibition on the risk of diabetes has been investigated using Mendelian randomisation approaches. One such study, which analysed data from 14 clinical trials and >112,000 patients, suggested a potential link between PCSK9 variants and an increased risk of diabetes (odds ratio: 1.07; 95% CI: 1.00–1.13).17 However, it is important to note that PCSK9 inhibitors are monoclonal antibodies that bind extracellular PCSK9 and may not have the same biologic effect as PCSK9 variants that lower LDL-C levels. Interestingly, in a pooled analysis of 10 clinical trials from the ODYSSEY Phase III programme in patients without diabetes at baseline, there was no effect of alirocumab on the incidence of new-onset diabetes or pre-diabetes.18 To date, several CV outcomes trials have been initiated to determine whether PCSK9 inhibitors confer any CV benefits, including the recently completed FOURIER trial (evolocumab),19 the ongoing ODYSSEY Outcome trial (alirocumab),20 and the discontinued SPIRE-121 and SPIRE-222 trials (bococizumab). Initial results from FOURIER demonstrate that evolocumab, in combination with statin therapy, significantly reduces the risk of CV events (defined as a composite of CV death, myocardial infarction, stroke, hospitalisation for unstable angina, or coronary revascularisation) compared with placebo (HR: 0.85; 95% CI: 0.79–0.92; p<0.001), in patients with ASCVD.19 While subtle differences exist between the designs of FOURIER and ODYSSEY (e.g., the primary endpoint in ODYSSEY is a composite of coronary heart disease death, myocardial infarction, ischaemic stroke, and hospitalisation for unstable angina), these trials will provide evidence regarding how to optimise the use of PCSK9 inhibitors in patients with high CV risk.
New Lipid Lowering Strategies in Diabetes: Insights from the ODYSSEY DM Programme
Doctor Lawrence A. Leiter
Key Points
- The ODYSSEY DM programme assessed the efficacy and safety of alirocumab in two groups of very high-risk populations with diabetes: patients on insulin therapy and patients with mixed dyslipidaemia.
- The ODYSSEY DM-INSULIN and ODYSSEY DM-DYLIPIDEMIA studies demonstrated the superior lipid lowering efficacy of alirocumab versus usual care and detected no new safety concerns.
- Together, these data support the use of alirocumab as a treatment option in people with diabetes and high CV risk.
The ODYSSEY Phase III programme was designed to evaluate the efficacy and safety of alirocumab in patients with hypercholesterolaemia and high CV risk, of whom approximately 30% had diabetes. Evidence from up to 104 weeks of follow-up in these Phase III trials demonstrated no effect of alirocumab on glycaemic control. The ODYSSEY DM programme was designed to further explore the efficacy and safety of alirocumab in patients with diabetes and comprised two pivotal studies: ODYSSEY DM-INSULIN23 and ODYSSEY DM-DYSLIPIDEMIA.24
ODYSSEY DM-INSULIN: Rationale and Key Clinical Data
Patients with Type 1 diabetes mellitus are often under-represented in lipid-lowering clinical trials and insulin-treated patients with Type 2 diabetes mellitus represent a cohort with long-standing disease and an increased risk of ASCVD. Furthermore, it is important to understand how alirocumab (a monoclonal antibody) behaves when coadministered with the biologic insulin.
DM-INSULIN assessed the efficacy and safety of alirocumab versus placebo in insulin-treated patients with Type 1 or Type 2 diabetes mellitus (plus high CV risk and above-target LDL-C levels despite maximum tolerated doses of statin therapy).23,25
DM-INSULIN was a randomised, double-blind, placebo-controlled study.23 Patients were aged ≥18 years with insulin-treated Type 1 or Type 2 diabetes mellitus (≥12 months), HbA1c levels <10%, LDL-C levels ≥1.81 mmol/L (70 mg/dL), ASCVD and/or at least one additional CV risk factor, and receiving a stable maximum tolerated dose of statin with or without other lipid-lowering therapies.23 Key exclusion criteria included triglyceride levels >4.5 mmol/L (400 mg/dL) and insulin treatment <6 months in duration or a stable insulin regimen/dose <3 months in duration.23 After screening, patients were randomised 2:1 to alirocumab (75 mg subcutaneously every 2 weeks [Q2W]) or placebo, stratified by diabetes type, for a 24-week treatment period with an 8-week safety extension. Alirocumab doses were adjusted at Week 12 to 150 mg Q2W if lipid targets (LDL-C levels <1.81 mmol/L [70 mg/dL]) were not achieved at Week 8. Patients remained on a stable diet for glucose and lipid management and received a stable dose regimen of statin and/or other lipid-lowering therapy throughout the study. The primary endpoint was percentage change from baseline in calculated LDL-C at Week 24, and safety up to Week 32.23
A total of 517 patients were randomised to treatment, 441 of whom had Type 2 diabetes mellitus. Of these patients, 294 were allocated to alirocumab and 147 to placebo.2 Baseline characteristics of the Type 2 diabetes mellitus population were generally balanced between treatment arms; the mean age was 64.0 years, mean duration of diabetes was 16.0 years, and mean HbA1c was 7.5%.2 Approximately 75% of patients were on statins, the majority of whom were receiving a moderate-intensity dose, and approximately 25% were statin intolerant.2 Baseline lipid profiles were comparable between treatment arms; calculated mean LDL-C was 2.9 mmol/L (110.8 mg/dL) in the alirocumab arm and 2.8 mmol/L (109.6 mg/dL) in the placebo arm.
At Week 24, alirocumab was associated with a significant reduction from baseline in LDL-C versus placebo (-48.2% versus +0.8%; p<0.0001), an effect that was achieved in most patients with the lower alirocumab dose (79.8% of patients were receiving 75 mg Q2W at Week 12).2 This finding was consistent with observations from the overall ODYSSEY programme (including pooled analyses) which reported LDL-C lowering ranging from -43.4 to -60.4%.26-28 Alirocumab also significantly reduced non-HDL-C levels versus placebo (least squares [LS] mean difference: -38.7%; p<0.0001), and produced significant reductions in apolipoprotein B and lipoprotein(a), and elevations in HDL-C levels.2 Glycaemic-related parameters, including HbA1c, fasting plasma glucose, and total daily insulin dose, were consistent between treatment arms throughout the study.2 Alirocumab demonstrated an acceptable safety and tolerability profile. The incidence of treatment-emergent adverse events was comparable between alirocumab and placebo arms (66.9% versus 66.2%, respectively). Allergic drug reactions were low in both treatment arms; 3.2% of alirocumab-treated patients had low-titer persistent anti-drug antibodies.
ODYSSEY DM-DYSLIPDEMIA: Rationale and Key Clinical Data
Individuals with diabetes and mixed dyslipidaemia are at high CV risk, yet alirocumab had not been specifically evaluated in this population prior to the DM-DYSLIPIDEMIA study. Furthermore, no previous trial of a PCSK9 inhibitor has used non-HDL-C as its primary endpoint.29 This study evaluated alirocumab versus lipid-lowering usual care in patients with Type 2 diabetes mellitus and mixed dyslipidaemia for those at high CV risk with below-target non-HDL-C levels despite maximum tolerated doses of statin therapy.24
ODYSSEY DM-DYSLIPIDEMIA was a randomised, double-blind, placebo-controlled study.24 Patients had Type 2 diabetes mellitus, non-HDL-C levels ≥2.59 mmol/L (100 mg/dL), triglyceride levels ≥1.70 and <5.65 mmol/L (150–500 mg/dL), and ASCVD or other CV risk factors.24 Patients were randomised 2:1 to alirocumab treatment (75 mg subcutaneously Q2W) or usual care for 24 weeks followed by an 8-week safety extension.24 Usual care permitted the optional addition of one of the following to statin therapy, ezetimibe, fenofibrate, omega-3 fatty acids, or nicotinic acid. Alirocumab doses were adjusted at Week 12 to 150 mg Q2W if lipid targets (LDL-C levels <1.81 mmol/L [70 mg/dL]) were not achieved at Week 8. Patients remained on a stable diet for glucose and lipid management, and received a maximum tolerated dose of statin therapy (or no statin if intolerant) throughout the study.24 The primary endpoint was percentage change from baseline in non-HDL-C at Week 24.24
A total of 276 patients were randomised to alirocumab treatment and 137 to usual care.3 Baseline characteristics were comparable between treatment arms; mean age was 63.5 years and mean HbA1c was 7.1%. Approximately 34% of patients had ASCVD while the remaining 66% had ASCVD plus additional CV risk factors. Lipid profiles were comparable between treatment arms; mean non-HDL-C was 4.0 mmol/L (155.1 mg/dL) with alirocumab and 4.2 mmol/L (161.5 mg/dL) with usual care.3
This important clinical trial will illuminate potential mechanisms and treatment strategies in patients with mixed dyslipidaemia or elevated atherogenic remnant cholesterol levels. For example, this trial will explore the clinical value of increasing LDL-receptor activity or hepatic catabolism of atherogenic lipoproteins for the lipoprotein phenotype with alirocumab versus usual care and fenofibrate, the latter mainly inhibiting synthesis of triglyceride-rich lipoproteins.
Details of the EASD scientific session on September 14th reporting new data from the ODYSSEY DM programme in patients with Type 1 and Type 2 diabetes mellitus were announced.30
Dual Sodium-Glucose Cotransporter 1 and 2 Inhibition: Of Mechanisms and Men
Professor Thomas Danne
Key Points
- Sotagliflozin is an oral, potent, dual inhibitor of the insulin-independent SGLT1 and SGLT2 transporters.
- Evidence from Phase II studies demonstrate that sotagliflozin, in combination with metformin, lowers HbA1c in patients with Type 2 diabetes mellitus.
- In addition, sotagliflozin therapy provides significant reductions in body weight and systolic blood pressure with an acceptable safety profile.
- Furthermore, the efficacy of sotagliflozin is maintained even in patients with low estimated glomerular filtration rate (eGFR) levels.
The book ‘Of Mice and Men’, a depiction of the American Dream, was first published by John Steinbeck in 1937. Seventy years later, in 2007, the Nobel Prize in Physiology and Medicine was awarded for creation of the first knockout mouse model. Today, results of emerging novel therapeutic agents for the treatment of Type 1 diabetes mellitus, a dream for many patients since the initial discovery of insulin, are being presented. These novel treatments are also a story of mice and men, as the therapeutic principle has been developed with the help of knockout mouse models and investigational agents have now completed Phase III trials in humans. The original principle was discovered 200 years ago when phlorizin, a glycoside and dual inhibitor of the insulin-independent sodium-glucose cotransporters SGLT1 and SGLT2, was first isolated by French scientists in 1835 from the bark of an apple tree.31 In subsequent years, understanding of phlorizin’s mechanism of action and clinical effects advanced significantly. Although phlorizin was shown to improve glycaemic control in diabetic animals, gastrointestinal (GI) side effects and rapid GI metabolism after oral administration prevented its development as an oral antidiabetic agent.32,33 Clinical studies have demonstrated the beneficial effects of SGLT2 inhibition on glucose homeostasis (Figure 1).31
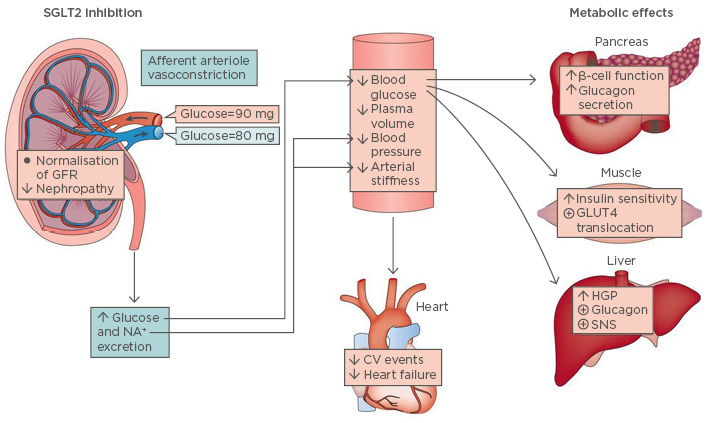
Figure 1: Physiological effects of sodium-glucose cotransporter 2 inhibition.
CV: cardiovascular; GFR: glomerular filtration rate; GLUT4: glucose transporter Type 4; HGP: hepatic glucose production; SGLT2: sodium-glucose cotransporter; SNS: sympathetic nervous system.
Adapted from DeFronzo et al.31
However, SGLT2 inhibition can be linked with an increased risk of diabetic ketoacidosis (DKA) and uncharacteristically mild-to-moderate glucose elevations (euglycaemic DKA).34 Multiple factors can trigger the onset of DKA, including insulin dose reductions and intercurrent illness. However, euglycaemic DKA is detectable and can be managed with proper patient education.35,36 Preliminary evidence indicates that dual inhibition of both SGLT1 and SGLT2 may serve as a strong target for diabetes management. Indeed, oral glucose tolerance tests have demonstrated reduced glucose excursions in both SGLT1 and SGLT2 knockout mice fed a high-fat diet versus wild- type controls.37
Sotagliflozin Mechanism of Action
Sotagliflozin, an investigational oral agent, provides dual inhibition of both SGLT1 and SGLT2 and thus differs from existing therapies which are selective for SGLT2. Sotagliflozin provides potent inhibition of SGLT1 and ~18x more potent inhibition of SGLT2 than canagliflozin.38 Predominantly expressed in the intestine, SGLT1 acts as the major intestinal glucose and galactose transporter, while SGLT2, which is expressed in the liver, facilitates reabsorption of filtered glucose.39,40 Therefore, dual SGLT1 and SGLT2 inhibition with sotagliflozin has the potential to lower postprandial glucose, and produce robust reductions in HbA1c with reduced renal glucose excretion that is maintained with reduced kidney function.41 In patients with Type 2 diabetes mellitus, SGLT1 is overexpressed in the GI tract, similar to the overexpression of SGLT2 in the kidney. Therefore, partial SGLT1 inhibition may provide additional benefits to patients that could not be achieved with SGLT2 inhibition alone.
Insights from Sotagliflozin Phase II Data in Patients with Type 2 Diabetes Mellitus
The safety and efficacy of oral sotagliflozin was evaluated in a Phase IIa, randomised, double-blind, placebo-controlled trial in 36 patients with Type 2 diabetes mellitus.42 At Week 4, sotagliflozin at doses of 150 mg/day and 300 mg/day were associated with significantly greater reductions from baseline in HbA1c versus placebo (-1.15% and -1.25% versus -0.49%, respectively).42 In addition, sotagliflozin, at both doses studies, was associated with incremental improvements in postprandial glucose compared with placebo. Furthermore, both doses of sotagliflozin were associated with increased levels of the glucagon-like peptide-1 (GLP-1) as indicated by an increase in total GLP-1 area under the curve.42 A 12-week, dose-ranging, Phase IIb study evaluated escalating doses of sotagliflozin (75, 200, and 400 mg once-daily; or 200 mg twice-daily) versus placebo in patients with Type 2 diabetes mellitus receiving metformin.43 Analyses of Week 12 data demonstrated that while HbA1c change from baseline was dose-dependently greater with sotagliflozin versus placebo, urinary glucose/creatinine ratios were not increased with higher sotagliflozin doses. This increased efficacy without increased urinary glucose excretion at 400 mg sotagliflozin was consistent with dose-dependent SGLT-1 inhibition above 200 mg, which differs from observations with selective SGLT2 inhibitors.43
Compared with placebo, sotagliflozin was associated with significant reductions in body weight at Week 12 with all doses tested (p≤0.001). Furthermore, sotagliflozin at higher doses (200–400 mg) significantly reduced systolic blood pressure compared with placebo (p≤0.017).43 The incidence of treatment-emergent adverse events was >3% in all sotagliflozin-treated patients, regardless of causality, which was consistent with the placebo arm (57.6–66.7% versus 66.7%, respectively). Nausea events were numerically higher with sotagliflozin 400 mg once-daily versus placebo (6 versus 3, respectively).43 Genitourinary events were low among both treatment arms, but numerically higher in sotagliflozin-treated patients versus placebo-treated patients (11 events were reported in total; 10 with sotagliflozin and 1 with placebo).43 In a separate study, the efficacy and safety of sotagliflozin versus placebo was evaluated in a 7-day study of patients with Type 2 diabetes mellitus and renal impairment (defined as an eGFR <60 mL/min/1.73m2).44 At Day 7, sotagliflozin treatment was associated with significantly greater postprandial glucose excursions compared with placebo in both the total population (p=0.003 versus placebo), and the subgroup of patients with eGFR <45 mL/min/1.73m2 (p=0.002).44 Furthermore, sotagliflozin compared with placebo significantly lowered systolic blood pressure (LS mean difference: -11.4; p=0.045), and numerically lowered diastolic blood pressure (LS mean difference: -4.5; p=0.08) in the subgroup of patients with eGFR <60 mL/min/1.73m2. Taken together, these data indicate that sotagliflozin is efficacious and well-tolerated in patients with Type 2 diabetes mellitus, including those with reduced renal function.
Unmet Needs in Adult Patients with Type 1 Diabetes Mellitus that Could be Addressed with an Oral Agent and the Sotagliflozin Clinical Development Programme
Doctor Anne Peters
Key Points
- Insulin therapy alone is often inadequate in patients with Type 1 diabetes mellitus, who may benefit from adjunctive treatments.
- Measurement of outcomes for adults with Type 1 diabetes mellitus should not be based solely on changes in HbA1c.
- The ongoing inTandem Phase III clinical programme will provide valuable insights regarding the potential role of dual SGLT1 and SGLT2 inhibition in the treatment of Type 1 diabetes mellitus.
Dual inhibitors of SGLT1 and SGLT2 could potentially represent an effective adjunct treatment to insulin therapy in Type 1 diabetes mellitus. Metabolic control varies with advancing age among patients with Type 1 diabetes mellitus. Evidence from the T1D Exchange clinic registry indicates that overall mean HbA1c levels range from 8.1–8.3% in childhood, increasing to >9.0% in adolescents (e.g., 17–20 years), gradually declining to ~7.5–7.8% in patients aged >30 years, and then modestly decreasing to <7.5% in individuals aged >65 years.45 Additional analyses from the registry demonstrated that most patients (up to 86%) were unable to achieve their HbA1c targets solely with insulin, an observation that was consistent across age groups.45 The inadequacy of insulin therapy alone has also been highlighted by other studies. One such study evaluated the effect of intensive versus conventional insulin therapy (≥3 versus 1–2 injections per day, respectively) on the incidence of CV disease over 30 years in patients with Type 1 diabetes mellitus.46 Analyses revealed that the incidence of hypertension increased with age, irrespective of whether patients received intensive therapy or conventional insulin therapy.46 Registry data on BMI among patients with Type 1 diabetes mellitus indicate that the proportion of patients with a BMI in the normal range decreases with age, and conversely, the proportion of patients with a BMI in the overweight or obese range increases with age, irrespective of insulin treatment.45
Improving Outcomes in Type 1 Diabetes Mellitus Beyond Glycated Haemoglobin
Difficulties in managing diabetes can have serious psychological consequences. Patients may develop diabetes distress, a state in which they can experience feelings of powerlessness (i.e. “my disease is out of control”), physician distress (i.e. disappointment with healthcare providers), and negative social perceptions (i.e. concerns around negative judgement from others).47 The path to glycaemic control is individual to each patient and more complex than the simple metric of HbA1c can measure. An estimate of mean HbA1c based on a measurement cannot provide an accurate report of daily fluctuations in glucose control and may potentially ‘mask’ episodes of significant dysglycaemia that can impact clinical outcomes and patient quality of life. In the USA, a number of clinical societies (AACE, American Association of Diabetes Educators [AADE], ADA, Endocrine Society, Pediatric Endocrine Society [PES], JDRF International, Helmsley Charitable Trust, and the T1D Exchange) are currently working together to establish the ‘Standardizing Clinically Meaningful Outcome Measures Beyond HbA1c for Type 1 Diabetes’ consensus statement, which will include definitions of standardised measures of outcomes such as hypoglycaemia and hyperglycaemia (by level of severity), time in glycaemic range, and DKA.
Selective Sodium-Glucose Cotransporter 2 Inhibition and Sotagliflozin in Type 1 Diabetes Mellitus
Selective SGLT2 inhibition offers an additional therapeutic option for patients with Type 1 diabetes mellitus; however, SGLT2 inhibitors should be used with caution in those patients who may be susceptible to DKA.35 A Phase II, placebo- controlled, proof-of-concept study evaluated the effects of dual SGLT1 and SGLT2 inhibition with sotagliflozin over 29 days in patients with Type 1 diabetes mellitus.48 At the end of the treatment period, change from baseline in HbA1c was significantly lower with sotagliflozin versus placebo (-0.55% versus -0.06%, respectively; p=0.02).48 Notably, this robust HbA1c-lowering effect of sotagliflozin versus placebo was not accompanied by an increase in hypoglycaemia. Analysis of continuous glucose monitoring data revealed that patients treated with sotagliflozin versus placebo spent a significantly greater proportion of time within the target glycaemic range of 3.8–10 mmol/L (70–180 mg/dL). In addition, there was no increase in the proportion of time spent in the below target range of <3.8 mmol/L (<70 mg/dL) with sotagliflozin versus placebo (Figure 2).48
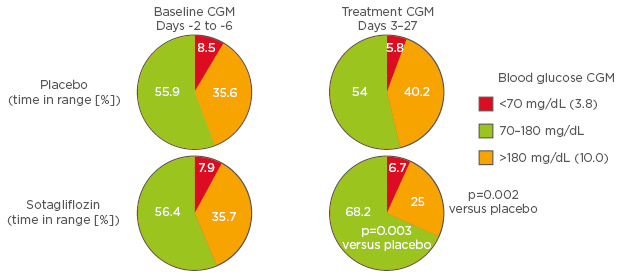
Figure 2: Physiological effects of sodium-glucose cotransporter 2 inhibition.
CGM: continuous glucose monitoring.
Adapted from Sands et al.48
Sotagliflozin inTandem Clinical Programme
The sotagliflozin inTandem Phase III clinical programme comprised three pivotal trials, inTandem1, inTandem2, and inTandem3.49,50 inTandem1 and inTandem2 were randomised, placebo-controlled studies that assessed the efficacy and safety of sotagliflozin (200 or 400 mg once-daily) on a background of optimised insulin, in patients with Type 1 diabetes mellitus. inTandem1 enrolled 793 patients from 79 sites in North America, while inTandem2 enrolled 782 patients from 99 sites across Europe and Israel.49,50 The studies consisted of a 24-week treatment period followed by a 28-week extension. The primary endpoint was change from baseline in HbA1c at Week 24. inTandem3 was a randomised, placebo-controlled trial which evaluated the efficacy and safety of sotagliflozin 400 mg once-daily on a background of standard of care insulin (i.e. not optimised) in 1,405 patients with Type 1 diabetes mellitus from 19 countries worldwide.51 The study comprised a 2-week screening period, 2-week run-in, and 24-week treatment period. The primary endpoint was the proportion of patients with HbA1c <7.0% and no episode of severe hypoglycaemia or DKA at Week 24. Results from the sotagliflozin inTandem Phase III clinical programme will provide valuable insights regarding the potential role of dual SGLT1 and SGLT2 inhibition in the treatment of Type 1 diabetes mellitus.
Enriching Diabetes Care: Technology and New Models for Intervention Strategies
Doctor Boris Kovatchev
Key Points
- Innovations in metabolic modelling and technology are revolutionising diabetes care.
- Optimisation of diabetes treatment is dependent upon achieving strict glycaemic control without increasing the risk of hypoglycaemia.
- The development of new diabetes-related technologies, such as closed-loop systems, follows three key steps: 1) formulation of the problem, 2) understand the system, 3) and control diabetes.
- Future integration of patient-level information (e.g., genetic profiling, laboratory results, and real-time monitoring) would create a diabetes treatment ecosystem that could potentially bring precision medicine into the care of people with diabetes.
Since the discovery of insulin in 1921, diabetes-related technology has progressed remarkably. Notable innovations have included the development of insulin pump systems, sensitive glucose monitoring techniques, and advanced metabolic assessment procedures;52 technological advances that together are helping to advance diabetes treatment. However, clinical optimisation of diabetes therapy (i.e. aiming to achieve and maintain strict glycaemic control without increasing the risk of hypoglycaemia) continues to represent a significant challenge for both physicians and patients.53 Optimisation of therapy can be achieved by developing new medications or technologies. The development of new diabetes technology follows three key steps: 1) formulate the problem quantitatively, 2) understand the metabolic system, and 3) control diabetes.
Formulate the Problem, Quantitatively
Optimisation of diabetes therapy can only be achieved through lowering glucose variability.54 A common limitation of traditional glucose variability measures is bias towards hyperglycaemia. This is due to the asymmetric nature of the blood glucose scale and that deviations towards hyperglycaemia occupy a wider range of the scale than deviations towards hypoglycaemia.54 Furthermore, the clinical risk associated with glycaemic excursions must differentiate between excursions into hyperglycaemia versus acute hypoglycaemia. Risk analyses of blood glucose data provides a means of quantifying glucose variability more accurately. These demonstrate that falling blood glucose levels are associated with a sharp increase in risk, while rising blood glucose values are associated with a gradual increase in risk (Figure 3).54,55 Accordingly, excursions into the range of extreme hypoglycaemia or hyperglycaemia are allocated progressively increasing risk values. Variance within the optimal euglycaemic range is attenuated, which reduces noise during data analysis.
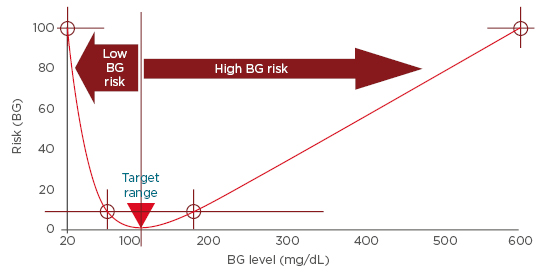
Figure 3: Risk analysis of blood glucose data.
BG: blood glucose.
Adapted from Kovatchev.54
Understand the System
The development of new medications and technologies is based on an in-depth understanding of the functioning of the human glucose control network in healthy individuals and in patients with Type 1 and Type 2 diabetes mellitus. Glucose homeostasis is regulated by a complex interplay of ‘intertissue communication’ between the pancreas, liver, gut, brain, and muscle, which is facilitated by glucose and its metabolites and is subject to environmental factors, specifically diet and exercise.56 In Type 2 diabetes mellitus, altered communication between tissues and an inability to adapt to changing metabolic states both play a critical role in the altered glucose homeostasis that fuels disease progression. The incretin effect represents an example of how glucose homeostasis is perturbed in patients with Type 2 diabetes mellitus. Defined as the difference in insulin secretory response elicited by oral glucose load versus intravenous glucose administration, the incretin effect is substantially diminished in patients with Type 2 diabetes mellitus.57
In addition, to a detailed knowledge of the human glucose control network, it is pivotal to fully understand the mechanism and clinical effects of diabetes treatments. Clinical studies provide quantitative data on the differential effects of various therapeutic approaches (e.g., basal insulin monotherapy versus GLP-1 receptor agonists versus a combination of both) on diabetes outcomes. Taken together, such understanding provides a comprehensive framework for the development of in silico metabolic models. These models enable realistic computer simulation of the metabolic manifestations of diabetes and of their treatment. In a multinational study, closed-loop control was compared to state-of-the-art open-loop therapy in adults with Type 1 diabetes mellitus, where the design of the closed-loop control algorithm was done in silico.58 Computer simulated experiments were used to generate data from 300 virtual subjects with Type 1 diabetes mellitus, from three distinct age groups who could be screened, measured, and treated individually. In silico modelling resulted in rapid (<6 months compared with the equivalent years of animal trials) and cost-effective system development and testing, leading to regulatory approval in multiple markets.58 In the USA, the U.S. Food and Drug Administration (FDA) has accepted in silico modelling for assessing human glucose and insulin utilisation, interstitial sensor performance, and subcutaneous insulin delivery.58 Furthermore, in silico models are now becoming an accepted alternative to animal trials for the preclinical testing of new insulin treatment strategies and artificial pancreas algorithms.58
Control Diabetes
The artificial pancreas represents the ultimate technological treatment of diabetes and has advanced considerably since the first devices were tested around 40 years ago. Early devices were impractical for outpatient use due to the intravenous route of glucose sensing and insulin infusion. However, they validated the feasibility of external glucose control, paving the way for future developments. The subsequent development of minimally invasive subcutaneous glucose sensing technology revolutionised closed-loop control systems. Modern systems comprise a continuous glucose sensor, insulin pump, and a sophisticated control algorithm that uses a mathematical model of the metabolic system to provide automated insulin delivery.59 The first portable closed-loop control system was introduced by the University of Virginia, Virginia, USA in 2011. The technology, which is controlled by a smart phone, links wirelessly to the glucose sensor and insulin pump to provide optimised, automated insulin delivery.60 To date, the effectiveness of several closed-loop control systems, including the Medtronic MiniMed 670G System, the Dexcom G4 with Software 505 + Roche insulin pump, and the Dexcom G4 Platinum + two Tandem t:slim insulin pumps have been evaluated in the outpatient setting.61-63 Time within range analyses demonstrated that closed-loop systems provided effective glycaemic control; patients were within the target glycaemic range of 3.8–10.0 mmol/L (70–180 mg/dL) >70% of the time and were below the target range <3% of the time. Time spent at very low blood glucose levels (<2.8 mmol/L [50 mg/dL]) was negligible (<0.4%).61-63 In addition, data from a recently completed ski camp study demonstrated that even during challenging winter-sport conditions, overall time within glycaemic range and time within range at night (3.00–7.00 am) was higher with closed-loop systems (71.3% and 84.6%, respectively) versus control (64.7% and 66.2%, respectively). Importantly, time below range was lower with closed-loop systems versus control (1.8% versus 3.2%, respectively).64
New Models for Intervention
Future therapeutic interventions could be derived from a diabetes treatment ecosystem. Such an ecosystem would bring together patient-level information, including genetic profiling, laboratory results, real-time monitoring, and predictive analytics, into a comprehensive virtual image of the patient, which would then allow treatment approaches to be tested efficiently in silico and tailored to each person.
Integrating Diabetes Care
Professor David Kerr
Key Points
- There is a need for integration of diabetes care and technology (e.g., closed-loop insulin delivery systems and smart insulin pens) in order to improve outcomes for people with diabetes and their families.
- The development of sophisticated algorithms, informed by population-wide data and machine learning, could lead to initiatives that help predict and prevent negative clinical outcomes.
- Achieving integration of care requires new thinking beyond segmenting into Type 1 or Type 2 diabetes mellitus to better reflect personal needs and opportunities based on measurable metrics of success that are affordable, accessible, and understandable to all users.
Diabetes care needs to change before we are likely to see the introduction of personalised diabetes treatment ecosystems. In the USA alone, >20% of all patients with diabetes have very poor glycaemic control (HbA1c <9%).65 Registry data from >16,000 patients with Type 1 diabetes mellitus show that the majority of this population (>70%) are above ADA-recommended HbA1c targets, irrespective of age.45 There is also evidence of a racial divide, in which individuals from minority populations have poorer glycaemic control.65 Going forward, the concept of value in healthcare when assessing the potential impact of new therapies or devices can be quantitatively defined as the quality of care (i.e. achieving glycaemic targets etc.) plus the experience of care, divided by the cost. Integration of care offers an opportunity to enhance the clinical value of diabetes treatments, but this will require better and more in-depth understandings of the five determinants of health, as they affect individuals with diabetes. These five determinants include 1) genetics (e.g., race and ethnicity-specific effects on drug pharmacology); 2) biology (e.g., intra and inter-individual variations in drug absorption and duration); 3) behaviour (e.g., treatment concordance); 4) psychology (e.g., impact of depression, diabetes distress, and fear of hypoglycaemia); and 5) society (e.g., treatment access, costs, and non-traditional factors, such as environmental temperature and pollution). Current efforts to segment diabetes, e.g., arbitrarily dividing diabetes into Type 1 or Type 2, add little value with regard to clinical care and outcomes. Integrated care provides a means to stratify individuals in a more relevant manner based on their clinical profile, such as hypoglycaemia avoider, diabetes loather, high-cost individual, and insulin user.
Integrated care requires digital diabetes health technologies. Future technological innovation in diabetes care will also include new therapies such as 1) implantable drug delivery systems; 2) automated completely closed-loop systems using a variety of sensors and effectors to maintain physiological homeostasis; and 3) miniaturised, wearable, non-invasive glucose monitoring systems or long-term, implanted continuous glucose sensors. New miniaturised wearable sensors and implanted sensors are already beginning to be used to create systems that links users and their care teams to enable precision management of diabetes.
Preliminary efforts to integrate care are beginning to emerge. In clinical practice, areas exist in which innovation is needed and progression to integration is relatively simple. In an analysis of 2,000 local Latino patients with diabetes, only ~37% of individuals received two HbA1c tests within 12 months, the minimum number of tests recommended by national and international guidelines. These data highlight that the current process of HbA1c testing (co-ordinating a patient and physician for an appointment, performing a test, and communicating the results to the patient) is proving difficult in clinical practice. One potential approach to overcome this would be to issue a shared service based on geolocation technology that could match a patient with an appointment, when necessary. Integrated systems such as this could potentially contribute to improvements in diabetes management beyond the introduction of new therapies.
Natural progression of technology and the availability of population-wide data could lead to the development of computer systems with the ability to monitor whole diabetes populations and predict the possibility of negative outcomes in individual patients. Similarly, the development of sophisticated algorithms through machine learning may help prevent the occurrence of negative outcomes by alerting clinical teams to individuals who require an appropriate intervention. These data, together with deep learning, automated predictive analytics using blocks of increasing complexity could lead to the prevention, and possibly even cure, of diabetes.
The implementation of integrated care would revolutionise diabetes care; a concept that was illustrated by an animated, hypothetical clinical case. Future developments may permit patients to use virtual reality to learn how to initiate insulin using their smart pen. Furthermore, reminders, support, advice, and education could be provided to patients by a ‘home doctor’, in which the patient could interact with a virtual physician. The provision of 24-hour care could become a reality through linking smart pens and supporting devices (e.g., smart phones, smart watches) through the ‘Internet of Medical Things’ to a population-health, centrally located clinician. This individual, with the assistance of robots, would monitor data transmitted between devices and provide support to patients when they are unable to contact their local physician. For example, if a patient experiences an issue outside of their local physicians’ working hours, data can be transmitted to the population-health, centrally located clinician who will consider the information and then send a recommendation to the patient’s smart device. This recommendation (e.g., reduce insulin dose) will automatically transmit to the smart insulin pen, which will then prepare the recommended dose.
Achieving integration requires the following, 1) target population must be defined, 2) appropriate metrics of success must be defined, 3) the materials need to be understandable for all users, 4) the benefits of integration need to be sustainable in their return on investment, and 5) the technology needs to be affordable.
Conclusion
Innovations in diabetes care are not exclusive to therapeutic and technologic advances. Increasingly, researchers are looking beyond measures of glucose control to address non-glycaemic outcomes that are relevant to patients with diabetes. Such innovations include the availability of treatments that address the high CV risk that persists in patients with diabetes, despite optimised lipid-lowering therapy, the development of new therapies with novel mechanisms of action for managing Type 1 diabetes mellitus, and new technologies that will facilitate the future integration of care. Advances such as these should help to drive innovation within diabetes management. Furthermore, the integration of these advances into daily clinical practice should help to further evolve and improve patient care in the future.