Abstract
Triple-Negative Breast Cancer (TNBC) represents a heterogeneous disease that includes different subtypes and accounts for approximately 20% of all breast cancers (BC). TNBC is oestrogen receptor-negative, progesterone receptor-negative, and human epidermal growth factor receptor 2-negative. In addition, the androgen receptor is expressed in roughly 10–32% of TNBC cases. TNBC is characterised by worse outcomes, including higher risks of relapse and visceral crisis compared to other BC subtypes (especially during the first 2 years post BC diagnosis).
Programmed death-ligand 1 (PD-L1) is widely expressed on the surface of lymphocytes, monocytes, natural killer cells, macrophages, and some other cells. Moreover, PD-L1 expression has been explored in different types of cancer (e.g., malignant melanoma, non-small cell lung cancer, renal cell carcinoma, and colon cancer).
Due to limited treatment options for TNBC, there is an urgent need for the development of novel diagnostic and therapeutic strategies. To fulfil this unmet need, different approaches, including immunotherapy, have been investigated in clinical studies (with the goal of matching therapies with specific BC subtypes). This article discusses some diagnostic considerations relevant to patients with TNBC (focussing on advanced or metastatic disease). It summarises the recent clinical trials, investigating novel targeted immunotherapeutic agents (e.g., pembrolizumab and atezolizumab) for TNBC, and highlights important implications for both research and clinical practice.
INTRODUCTION
Despite the impressive advances that have been made in cancer diagnosis and therapy, there still exist groups of patients who are not responding to standard anticancer treatments. In particular, triple-negative breast cancer (TNBC) represents a difficult-to-treat, heterogeneous disease that includes different subtypes and accounts for approximately 20% of all BC.1 TNBC is oestrogen receptor (OR)-negative, progesterone receptor (PR)-negative, and human epidermal growth factor receptor 2 (HER2)-negative.1 In addition, androgen receptor (AR) is expressed in roughly 10–32% of TNBC.2 TNBC is characterised by worse outcomes, including higher risks of relapse and visceral crisis, compared to other BC subtypes (especially during the first 2 years post BC diagnosis).3 Because treatment options for TNBC are very limited, there is an urgent need for the development of novel therapeutic options associated with reliable diagnostic tests. There is a growing interest in targeting the immune system as part of BC therapy.4 According to the cancer immunoediting model, the immune system plays a dual role that consists of the host protection (via elimination of tumour cells) and the impact on the tumour (via editing its genome).5 In this context, using immune checkpoint blockers can potentiate immunoediting. This, in turn, may contribute to ‘shaping’ the tumour and enforcing T-cell-dependent immunoselective efforts (via the immune checkpoint blockade).5
Programmed death-ligand 1 (PD-L1) is an Ig superfamily haplotype Type I transmembrane glycoprotein (related to apoptosis).6,7 PD-L1 is widely expressed on the surface of lymphocytes, monocytes, natural killer (NK) cells, macrophages, and many other cells.7 Moreover, PD-L1 expression has been explored in different types of cancer, including malignant melanoma, non-small cell lung cancer, renal cell carcinoma, colon cancer, and oesophageal cancer.7 Similarly, programmed cell death protein 1 (PD-1), which is an inhibitory immune checkpoint that limits T-cell effector functions within tissues, is expressed on the surfaces of immune effector cells (such as T cells, B cells, NK cells, dendritic cells [DC], and many tumour infiltrating lymphocytes [TIL]).7 Recently, some novel immunomodulatory agents, including immune checkpoints inhibitors, have shown promising effects in subgroups of women with advanced or metastatic TNBC.8 For instance, increased PD-L1 expression on the surface of TNBC cells provides the target for such immunotherapeutic strategies. In particular, the PD-L1 inhibitor, atezolizumab, and the PD-1 inhibitor, pembrolizumab, have revealed beneficial results in recent clinical trials (Table 1).8
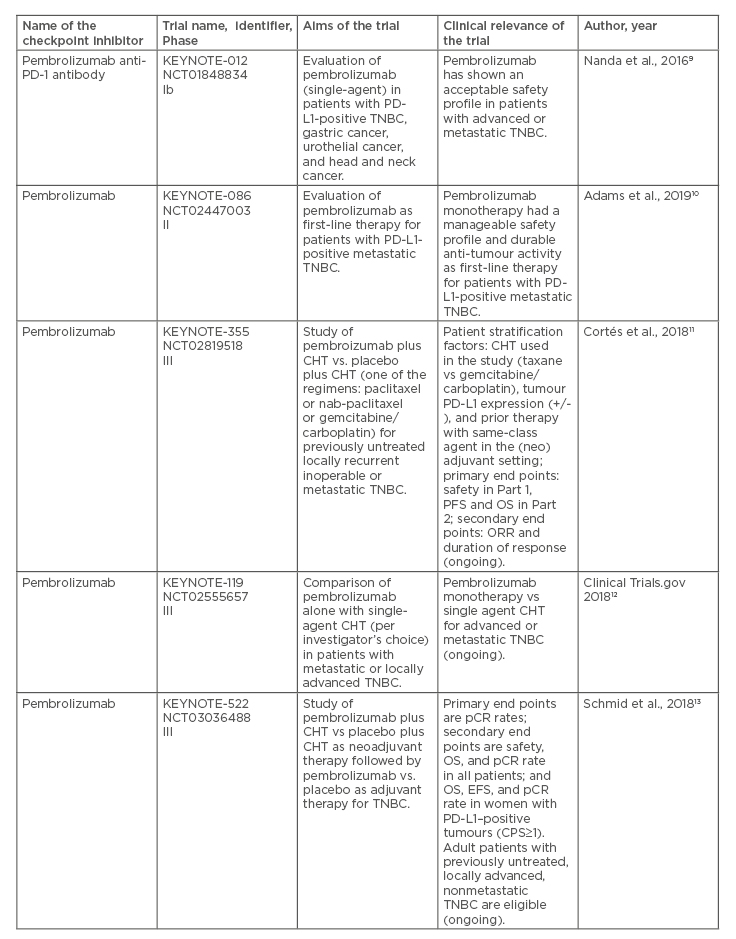
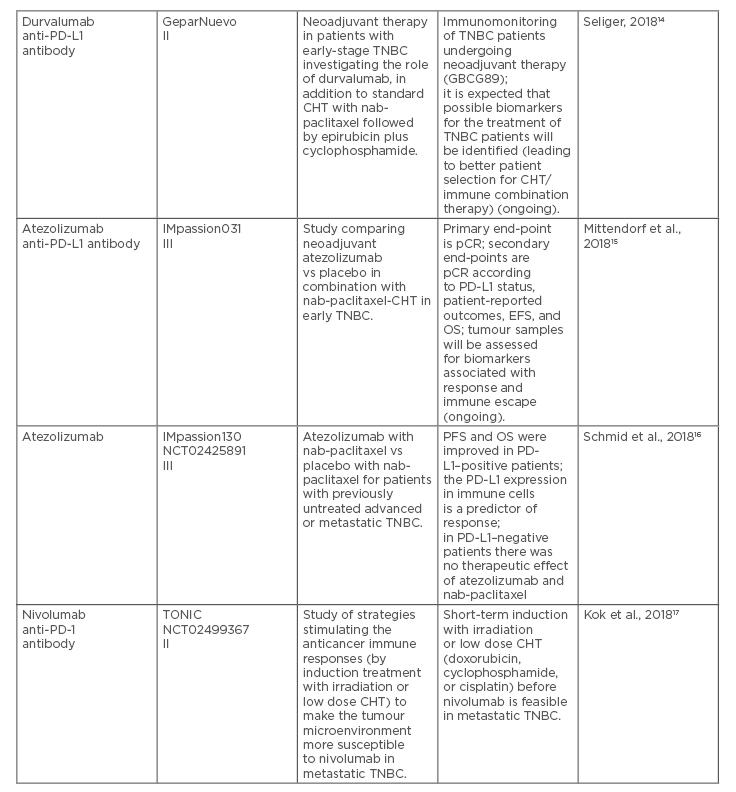
Table 1. Recent clinical trials on immune checkpoint inhibitors in patients with locally advanced or metastatic triple-negative breast cancer.
CHT: chemotherapy; CPS: combined positive score; EFS: event-free survival; IHC: immunohistochemistry; ORR: overall response rate; OS: overall survival; pCR: pathologic complete response; PD-1: programmed cell death protein-1; PD-L1: programmed death ligand 1; PFS: progression-free survival; TNBC: triple-negative breast cancer.
This mini review presents some novel diagnostic considerations related to patients with TNBC, focussing on advanced or metastatic disease. It summarises the main clinical trials leading to approval of immunotherapy (e.g., targeting the PD-1/PD-L1 pathway) for TNBC, and highlights important implications for further research and clinical oncology practice.
CANCER IMMUNOEDITING, IMMUNOLOGICALLY-RESPONSIVE, AND IMMUNOLOGICALLY-IGNORANT TUMOURS
Multiple molecular changes, which occur as a result of malignant tumour progression, should facilitate the distinction between cancer cells and healthy cells. Consequently, tumour cells should be recognised as foreign by the immune system, and subsequently destroyed. Unfortunately, tumours are seldom rejected spontaneously because of their capability to maintain an immunosuppressive microenvironment.18 In fact, the interplay between cancer cells and immune system cells (within the tumour microenvironment) creates the possibility for neoplastic cells to escape from immune surveillance.18 Based on the cancer immunoediting concept, the immune system (via interactions between tumour and host) recognises tumour-specific antigens, protects the host (by elimination of tumour cells), and ‘shapes’ the developing tumour (via editing the cancer genome).18 In this way, the tumour immunogenicity is
being reduced.18
At present, there is a need to explore the role of cancer immunoediting in the context of anticancer immunotherapy.19 For instance, anticancer immunotherapies with checkpoint inhibitors, such as anticytotoxic T lymphocyte-associated antigen 4 or anti-PD-1/-PD-L1 antibodies, have revealed some positive clinical responses.19 However, one of the greatest challenges is intrinsic resistance to immunotherapy and the development of resistant disease after therapy, i.e., an acquired resistance to immunotherapy.19 In an effort to address these patterns, anticancer immunotherapy, with the use of modern biomarkers, has emerged as a novel treatment modality for various, difficult-to-treat malignancies.19,20
In general, malignancies can be classified as immunologically-responsive or immunologically-ignorant.20 Immunologically-ignorant tumours are characterised by low mutation load, immune tolerance against self-antigens, and absence of infiltrating T cells.12 In contrast, immunologically-responsive tumours are characterised by the presence of numerous infiltrating T cells, which illustrate intrinsic T-cell immune-inhibition and extrinsic tumour-related T-cell immuno-suppression.22 The process of T-cell immune-inhibition is mediated via activation of immune checkpoint molecules (e.g., cytotoxic T lymphocyte-associated antigen 4, PD-1, T-cell immunoglobulin mucin-3, and lymphocyte-activation gene 3).19,21 This article will focus on the PD-1/PD-L1 checkpoints.
IMMUNE CHECKPOINTS – THEIR PHYSIOLOGICAL ROLE AND THERAPEUTIC POTENTIAL IN PATIENTS WITH CANCER
Physiologically, immune checkpoint molecules are ‘in charge’ of preventing tissue damage that occurs during infections and autoimmunological processes. Immune checkpoints are inhibitory receptors, which are mostly expressed on the surfaces of T cells and tumour cells where they mediate the interactions between these cells.23 In an adaptive immune resistance mechanism, the involvement of immune checkpoints on T cells by tumour cells suppresses the cytotoxic ability of T cells.24 This allows tumour cells to escape cytotoxicity and protects cancer from immune system attacks.24
T cell immune-inhibition decreases activity of cytotoxic T lymphocytes and reduces the recruitment of anti-inflammatory cells, regulatory T cells, and myeloid-derived suppressor cells.21,24 When PD-1 receptors on T lymphocytes are activated and bound to their relevant ligands PD-L1 and PD-L2, these immune checkpoints inhibit T-cell functions. In this way, the PD-1/PD-L1 axis is responsible for regulation of T-cell activation and prevention of tissue damage. On the other hand, however, the PD-1/PD-L1 axis enables tumour cells to evade immune surveillance.21,24
PD-L1 EXPRESSION ON THE TUMOUR CELLS AND TUMOUR INFILTRATING LYMPHOCYTES – RELATIONS WITH THERAPEUTIC EFFECTS
According to the concept supported by the results of the KEYNOTE-001 trial,12 related to immunotherapy for patients with cancer, it has been suggested that elevated tumour cell expression of PD-L1 correlates with immune system evasion. This, in turn, can lead to a worse prognosis among patients with malignancies treated with checkpoint inhibitors.25
However, based on a study of patients with cancer receiving therapy with anti-PD-L1 agents, in which two times as many patients with low or no PD-L1 expression on the tumour cells had beneficial clinical outcomes compared to the ones who had tumours with PD-L1 overexpression, it was revealed that this relation differed in various types of cancers.18,19
Additionally, according to a recent, large meta-analysis, which has addressed the expression of PD-L1 and prognosis in patients with BC, it has been revealed that PD-L1 positivity was ranging from 21 to 56%.26 Furthermore, it should be noted that in the majority of PD-L1-positive BC, PD-L1 expression was focal and limited to a small proportion of cancer cells, rather than diffuse.27
To date, TNBC has been viewed as ‘immunogenic’ (e.g., in terms of PD-L1 expression in both tumour and inflammatory cells, such as TIL).28 As a consequence, anti-PD-1/PD-L1-targeted therapies can be added to the TNBC treatment arsenal.28.29 In fact, TNBC has elevated PD-L1 expression, mostly in inflammatory (immune) cells and in some malignant cells.27,29 TIL can increase during both chemotherapy (CHT) and radiotherapy, and this may be caused by increased activation of CD8+ T cells and IFN-gamma secretion (during CHT or RT), which can stimulate PD-L1 expression.30 Elevated levels of TIL have been associated with improved disease-free survival and overall survival rates among TNBC patients.30 In addition, the presence of TIL in the breast tumour microenvironment may (to some degree) predict responses to neoadjuvant and adjuvant CHT.30 For instance, elevated numbers of TIL correlate with increased pathological complete responses (pCR) in patients with TNBC.30 Therefore, TIL play the role of prognostic and predictive markers of response to anticancer therapies.30
PD-1/PD-L1 AXIS AND BIOMARKERS OF RESPONSE TO PD-1/PD-L1 INHIBITION
PD-L1 overexpression in tumour cells may be considered a prognostic biomarker, but not a predictive biomarker due to different factors.25 For instance, PD-L1 expression may be influenced by TIL that produce IFN-gamma which, in turn, contributes to more beneficial clinical outcomes.25 Despite using various immunohistochemistry (IHC) staining methods, there is still no standard procedure for evaluation of PD-L1 expression.25 This is partially because of the fact that the PD-L1 heterogeneity reflects a dynamic process, in which a tumour may not express PD-L1 at baseline. It should be highlighted that TNBC has a higher level of PD-L1 expression, thus a blockade of PD-L1 with the use of novel immune checkpoint inhibitors can activate tumour-specific T-cell responses, leading to enhanced anti-tumour activity and better outcomes for this group of patients.31
An innovative application of the immune checkpoint inhibitors against either PD-1 or its PD-L1 have reshaped the therapeutic landscape of many difficult-to-treat malignancies, including TNBC.18,19,32 The interplay between PD-1 on T-cells and its ligands PD-L1 and PD-L2 on malignant cells causes T-cell exhaustion and leads to conversion of T effector cells to immunosuppressive T regulatory cells.32 In this scenario, the immune checkpoint inhibitors (acting against PD-1 or PD-L1) block the suppressor PD-1/PD-L1 axis. This leads to the reactivation of cytotoxic T effector cells and invigoration of the anticancer power of the immune system.32
IMMUNOLOGIC CONSIDERATIONS IN METASTATIC TNBC
It should be noted that metastatic BC represent microenvironmental systems, in which cell proliferation and apoptosis often coexist with immune system cell infiltration. Apoptotic tumour cells undergo phagocytosis, and tumour-specific antigens are expressed on the major histocompatibility complex molecules by tumour-infiltrating antigen presenting cells (APC). Subsequently, APC can activate antigen-specific cytotoxic T lymphocyte responses.
Under these circumstances, metastatic TNBC, which is positive for PD-L1, responds to a combination therapy with monoclonal anti-PD-L1 antibody (e.g., atezolizumab) and CHT (e.g., nab-paclitaxel).16 In this context, an agent such a nab-paclitaxel increases expression of tumour-specific antigens and invokes apoptosis, contributing to the antigen presentation via APC. In this way, suppressive signal inactivating T cells is stopped because the immune checkpoint inhibitor (e.g., atezolizumab) blocks the interaction between PD-1 and its ligands (PD-L1/2) to reverse T-cell suppression.32
INSIGHTS INTO THE IMPASSION130 TRIAL
The promising results of the randomised clinical trial (RCT) IMpassion130 have led to approval of atezolizumab (an anti-PD-L1 agent) in combination with CHT (using nanoparticle albumin-bound [nab] paclitaxel) for the therapy of patients with unresectable, locally advanced, or metastatic TNBC (Table 1)16. This approval was based on the Phase III RCT (atezolizumab plus nab-paclitaxel versus placebo plus nab-paclitaxel), involving >900 women with TNBC (with no previous treatment for the metastatic BC). The reason for using a combination of the checkpoint inhibitor with taxane-based CHT (which blocks mitosis) was that this therapy can increase the tumour-antigen release and augment anti-tumour responses to the immune checkpoint inhibition.33 It should be noted that in the IMpassion130 trial, prior to applying the atezolizumab therapy, tumour samples were evaluated by IHC for the presence of PD-L1 expression (using SP142 clone, Ventana, Roche, Switzerland).16 PD-L1 expression was assessed via the presence of tumour-infiltrating (immune) cells, using “a percentage of tumour area” <1% (meaning PD-L1 negative status) or ≥1% (meaning PD-L1 positive status).16 The IMpassion130 trial has shown that the patients whose cancers were positive for PD-L1 (roughly 41%) and received atezolizumab had better outcomes compared to the ones treated with nab-paclitaxel only (i.e., median progression-free survival was 7.2 months in the atezolizumab–nab-paclitaxel arm, compared to 5.5 months in the placebo-nab-paclitaxel arm).16 In the PD-L1-positive subgroup, the response rate was approximately 59% in the atezolizumab-nab-paclitaxel group, compared to approximately 43% in the placebo–nab-paclitaxel group.16 It should be pointed out that 10% of the patients in the atezolizumab–nab-paclitaxel group achieved a complete response, compared to only 1% of the ones in the placebo–nab-paclitaxel group.16 In addition, the atezolizumab plus nab-paclitaxel combination therapy arm has revealed a relatively good safety profile (i.e., the most typical adverse effects included hair loss, nausea, vomiting, diarrhoea, constipation, poor appetite, fatigue, tingling or numbness in the hands and feet, anaemia, cough, headache, and neutropenia).16
PD-L1 expression in both cancer cells and immune cells (as detected by IHC) represents a predictive biomarker according to the IMpassion130 trial.16 In addition, diagnostic antibodies have been validated as companion or complementary diagnostics. In accordance with the U.S. Food and Drug Administration (FDA) definition, companion diagnostics is a medical device that provides information that is essential for the safe and effective use of a corresponding drug or biological product. Similarly, complementary diagnostics is a test that aids in the benefit–risk decision-making about the use of the therapeutic product, in which the difference in benefit–risk proportion is clinically meaningful.34 It should be noted that SP142 (Ventana) represents the companion/complementary diagnostics not only for some subtypes of BC, but also for non-small cell lung cancer and bladder cancer.34 In fact, the recent approval of atezolizumab for the treatment of TNBC is applicable only to patients in whom BC express PD-L1, based on the Ventana diagnostic antibody SP142 test.16,34
FOCUS ON PD-L1 EXPRESSION ON THE IMMUNE CELLS INSTEAD OF THE TUMOUR CELLS
It should be noted that in the IMpassion130 trial, PD-L1 staining was focussed on PD-L1 expression on the immune cells, instead of the tumour cells (contrary to other types of cancer, such as the lung cancer).16 For instance, in the IMpassion130 trial 41% of patients were classified as PD-L1-positive, based on immune cells staining (at a cut-off of 1%).16 In addition, in this trial only 9% of patients were classified as PD-L1-positive on BC tumour cells, and most of them were also PD-L1-positive on the immune-cells. In fact, there may by some prognostic value (or a small difference in outcomes) in patients with PD-L1-positive expression, based on the immune versus tumour cell staining.16 In contrast, only approximately 2% of patients were PD-L1 positive on the tumour cells and PD-L1-negative on the immune cells. In addition, almost 60% of patients who were PD-L1 negative on the immune cells did not appear to derive substantial benefits from atezolizumab.16 Therefore, routine testing for PD-L1 status, among newly diagnosed patients with metastatic and locally advanced TNBC, should be merited to determine whether or not they can derive benefits from atezolizumab and nab-paclitaxel combination treatment.16 At this point, it is important to explain some key differences in the biology of PD-L1-positive and PD-L1-negative tumours. Moreover, making the PD-L1-negative tumours more immunogenic (e.g., via adding another agent, which may possibly alter their immunogenicity) can hopefully offer some therapeutic benefits.
FURTHER RESEARCH DIRECTIONS FOR IMMUNE CHECKPOINT INHIBITORS IN LOCALLY ADVANCED AND METASTATIC TRIPLE-NEGATIVE BREAST CANCER
In addition to the IMpassion130 trial, some important lessons have been learned from recent or ongoing clinical trials on immune checkpoint inhibitors in advanced or metastatic TNBC (Table 1).9-17 Continuous efforts are still necessary to optimise the therapy in the PD-L1-positive patients, and to design innovative approaches for the PD-L1-negative patients with TNBC. Due to remarkable progress in molecular characteristics of TNBC, not only immune checkpoint inhibitors, but also some other modern therapeutics, including poly ADP-ribose polymerase-1 inhibitors, tyrosine kinase inhibitors, androgen receptor inhibitors, and antibody-drug conjugates, are going to be explored in depth for this highly aggressive subtype of BC.35
It should be highlighted that it is essential to properly identify patients who may favourably respond to therapies with PD-1/PD-L1 checkpoint inhibitors. Moreover, in the dynamic interplay of the cancer cells with different immune cells (e.g., T lymphocytes, B lymphocytes, and APC), reliable biomarkers are needed to precisely predict the effects of the PD-1/PD-L1 checkpoint inhibitors among patients with TNBC. In addition to the PD-1/PD-L1 status, such biomarkers may include tumour mutational burden/load, microsatellite instability status, and the number of TIL.8,19,21,30,32,35
Further investigation in TNBC should also involve predictive and prognostic biomarkers for proper stratification of patients, who would be the most appropriate candidates for immune checkpoint inhibitor therapies.14,15,36 In addition, the optimal timing of administration and the best combination approaches (e.g., CHT, targeted therapy, and radiotherapy; administered concomitantly or sequentially) represent the main research questions that need to be addressed.35,36 Moreover, the treatment responses, survival outcomes, and safety issues, in monotherapy and in combination therapy should be investigated long term, in large-scale RCT. For instance, the anti-PD-1 and anti-PD-L1 monoclonal antibodies (e.g., pembrolizumab, durvalumab, atezolizumab, and nivolumab) are currently being tested in clinical trials among women with locally advanced or metastatic TNBC (in neoadjuvant or adjuvant settings) (Table 1).11-15,17 Furthermore, in the early TNBC setting, an assessment of the benefits of possible adding the immune checkpoint inhibitors to the neoadjuvant CHT is going to be explored.14,15,36 Simultaneously, studying communication networks between cancer cells and immune cells in the tumour microenvironment, as well as developing multi-modal management plans aimed at inducing anti-tumour immune responses, may hopefully improve clinical outcomes among patients with TNBC.37,38
CONCLUSION
Advances in cancer immunotherapy highlight the necessity of continuous learning about cancer immunology and the interactions of immune cells and tumour cells within the malignant tumour and its microenvironment. Novel immunotherapy strategies magnify the immune system actions and evoke durable tumour-specific immune memory. Consequently, some monoclonal antibodies that mediate the immune checkpoint receptors have provided promising improvements for patients with TNBC (e.g., in the metastatic setting).
Immune checkpoint blockade as monotherapy or combination therapy (e.g., atezolizumab and nab-paclitaxel) demonstrated some encouraging results as first-line therapy for metastatic TNBC (e.g., improvements in PFS, compared to CHT alone). The recent FDA approval of atezolizumab, a selective immune checkpoint inhibitor targeting PD-L1, plus nab-paclitaxel for the treatment of patients with PD-L1-positive, unresectable, locally advanced, or metastatic TNBC augments the therapeutic armamentarium for such a challenging BC subtype.