WHAT IS HISTOTRIPSY?
Histotripsy is a non-invasive, non-thermal, and non-ionising focused ultrasound (US) treatment method that destroys tissue through mechanical disruption caused by cavitation. Short-duration (<20 μs) high-pressure US pulses cause the rapid expansion and collapse of endogenous gas bubbles in targeted tissue.1,2 This results in high local mechanical stress and strain, eventually leading to rupture of cell membranes and liquification of tissue.3 Tissue destruction by histotripsy is a threshold effect in that a tissue-specific cavitation threshold needs to be reached before cavitation and tissue damage occur.4-6 Certain collagen-containing tissues with high mechanical strength, such as ureters, bile ducts, and large blood vessels, are relatively resistant to damage from histotripsy compared to highly cellular parenchymal organs and many tumours.7 Treatment zones are characterised by precise margins, no damage to intervening tissues, and acellular debris within the treatment zone that is rapidly resorbed.8
Histotripsy differs from high-intensity focused US thermal therapy due to the non-thermal (mechanical) nature of histotripsy and the threshold effect that increases precision, spares certain structures with high mechanical strength, and avoids tissue damage outside of treatment zones.4 Another potential benefit of histotripsy versus thermal methods is the post-treatment preservation of neo-antigens, which have the potential for immune stimulation.9-11 Histotripsy does not appear to increase the risk of parenchymal haemorrhage, even in anticoagulated subjects.12 To date, histotripsy has been applied in small clinical trials for the treatment of benign prostatic hyperplasia and malignant liver tumours,13,14 and larger trials are currently planned or underway. Multiple future applications are being actively explored in preclinical models and include treatment of benign and malignant tissue in the liver, kidney, pancreas, brain, thyroid, subcutaneous fat, and blood vessels.10,15-21
This manuscript focuses on the imaging surrounding histotripsy, a technique that uses microsecond pulses to generate inertial cavitation. Boiling histotripsy is a related technique that uses millisecond US pulses to generate boiling bubbles to liquefy target tissue.22,23 Boiling histotripsy is not yet in clinical use in humans, and the similarities and differences between the techniques are beyond the scope of this review.
Successful histotripsy treatment depends on accurate and precise image guidance. Diagnostic US is currently used to target and monitor histotripsy. The current histotripsy device being prepared for human clinical use consists of a diagnostic US transducer co-axially aligned with the larger therapeutic treatment head (Figure 1). Cavitation produced by the therapy transducer forms an echogenic focus in tissue (Figure 1), which enables the diagnostic transducer to be used for targeting, monitoring, and limited post-treatment assessment.
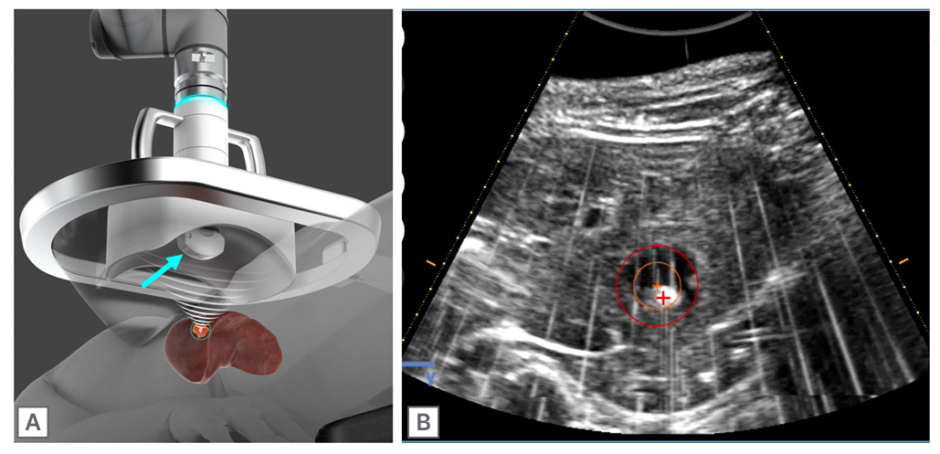
Figure 1: Histotripsy treatment head.
A) The therapeutic US transducer is co-axially aligned with a diagnostic US transducer (blue arrow), which can be used for planning and monitoring of the treatment in real time. B) The bubble cloud appears hyperechoic on B-mode US and is visible during histotripsy treatment of a healthy swine liver. During planning, the treatment zone is selected (red circle) and the bubble cloud is aligned to the crosshairs (red crosshair). Treated tissue within the red circle appears hypoechoic.
US: ultrasound.
MRI and conventional CT guidance cannot currently be used to guide histotripsy. The robotic arm, treatment head, diagnostic US transducer, and coupling water bath of current clinical systems will not fit into the bore of either conventional CT or MRI. In addition, the robotic arm and US transducer components are not currently MRI compatible. However, future development of both CT and MRI guided histotripsy systems is anticipated as components decrease in size and become MRI compatible. Fusion imaging between a pre-procedure CT or MRI and the co-axial diagnostic US transducer is possible; however, limitations such as tissue deformation from water bath compression, respiratory motion, and peristalsis may decrease the accuracy of fusion images.
ULTRASOUND GUIDANCE IN HISTOTRIPSY
US imaging provides many benefits for planning and monitoring histotripsy treatments. The co-axially aligned diagnostic transducer can be extended forward for direct contact with the body wall, which provides better image quality than a stand-off transducer in a water bath. Advantages of using US for targeting and monitoring histotripsy include real-time visualisation of the bubble cloud, multiplanar capability, and colour doppler imaging to visualise blood vessels. The fixed alignment of the therapeutic and imaging transducers is a particular advantage in that the focal zone of the therapeutic transducer remains in the field of view of the diagnostic transducer regardless of the position of the treatment head.
Cavitation bubbles created by histotripsy are echogenic on B-mode US and are an effective surrogate for cavitation forming in tissue (Figure 1). The visibility of the cavitation bubble cloud is useful for several stages of the planning and treatment process.
For treatment planning, a series of test pulses dispersed throughout the planned treatment zone are used to determine the minimum voltage required to create cavitation. During each test pulse, the system voltage is slowly increased by the user until the bubble cloud is observed on B-mode US, indicating that cavitation is occurring. This use of test pulses assures that the minimum amount of power necessary to create cavitation is used throughout the treatment and that heterogeneous tumours are adequately treated.24 Test pulses occur during the planning stage and do not affect the treatment time, which varies depending on the total volume to be treated. The visualisation of the bubble cloud can also be used for correction of the varying speeds of sound inherent in treating through the inhomogeneous body wall and intervening tissues.25
Post-treatment assessment is usually performed with either contrast-enhanced CT or MRI. However, treatment effects may also be detected by US due to histotripsy-mediated destruction of cell membranes and other echogenic tissue interfaces, which results in tissue liquefaction and hypoechoic transformation of tissue in the treatment zone.26
CHALLENGES OF ULTRASOUND FOR HISTOTRIPSY
Diagnostic US provides many benefits for real-time histotripsy visualisation as described above. However, intrinsic limitations in the ability to visualise some abdominal tumours with diagnostic US alone can be an important problem when targeting and monitoring histotripsy. While these tumours can be treated with histotripsy, isoechoic or deeply situated tumours, or those that are obscured due to obesity, overlying bowel gas, ribs, or lung, can be difficult to target. Tumours at the liver dome may also be challenging to visualise. US contrast agents may be used to help differentiate tumours from normal surrounding structures, but the safety profile of histotripsy in combination with bubble-containing agents is unknown, and, therefore, this approach is not recommended.
One important difference between conventional diagnostic US and US imaging during histotripsy is the amount of compression possible using each technique. While the diagnostic transducer can be extended to directly contact the abdominal wall, the robotic arm holding the treatment head has a limit to the amount of force that can be applied during scanning and treatment. This results in a limited ability to compress the abdomen to visualize deep or otherwise challenging targets.
CONE BEAM CT GUIDED HISTOTRIPSY
Cone beam CT (CBCT) offers an alternative or complement to US targeting. The open-bore C-arm architecture of most CBCT systems provides adequate clearance to contain the robotic treatment arm and water bath. The nature of CBCT provides both 2D fluoroscopic images for rapid adjustments and calibration during setup, as well as multi-planar volumetric CT scans for targeting. Unlike US, X-rays can be used to image through air, dense tissue, or depth, allowing for visualisation of targets through bone, lung, and bowel. Iodinated contrast can be safely used to visualise anatomy and differentiate tumours for targeting without adversely affecting histotripsy treatment. CBCT image quality is rapidly improving, but not yet at the level of conventional closed-bore CT scanners. However, the widespread availability, large number of experienced users, and familiar image format will likely aid in the rapid adoption of CBCT guided histotripsy.
Previously proposed CBCT targeting methods leverage the robotic arm for precise positioning of the treatment head based on a target and treatment angle selected on a volumetric CBCT scan (Figure 2).27 To this end, the spatial relationship between the robotic arm and the C-arm is determined, which is commonly referred to as hand-eye calibration. This can be accomplished during the setup of the system without the patient present by acquiring 2D fluoroscopic or 3D volumetric scans of the transducer head, or a dedicated calibration phantom attached to the robotic arm. These images can be used to determine the 3D position and orientation of the robotic arm attachment in the C-arm co-ordinate space and relate it to the known joint angles of the robotic arm.
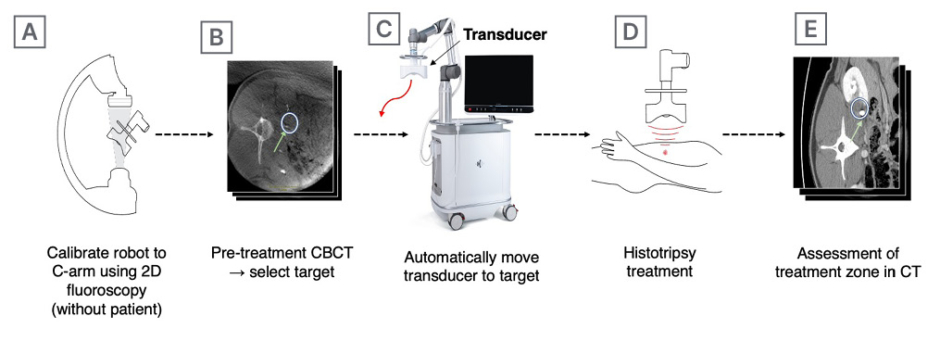
Figure 2: Cone beam CT-guided histotripsy.
To precisely target the kidney and ureter in a healthy swine model, a pre-treatment CBCT was obtained following the administration of IV contrast to opacify the ureter (B, green arrow). The treatment zone was selected (B, white circle) and the treatment head was automatically moved into position (C) to perform the treatment in (D). Follow-up CT (E) was performed immediately post-procedure with an additional bolus of IV contrast, with the treatment zone (white circle) and undamaged ureter (green arrow) visible.
CBCT: cone beam CT; IV: intravenous.
After the system is calibrated, the patient is positioned on the table and the water bath fixed over the targeted area. A volumetric 3D scan is taken using the C-arm to capture the target anatomy during compression from the water bath. The physician can select the target in the volumetric scan as well as adjust the orientation of the virtual treatment head, which is displayed in real-time with the volumetric scan. This allows for an opportunity to reduce the number of obstructions along the treatment path and avoid collisions with the patient. After the treatment plan is finalised, the robotic treatment arm automatically moves the histotripsy transducer in position to treat the selected area. The diagnostic US probe mounted inside the treatment head can be used to complement CBCT imaging if the target is visible.
POST-TREATMENT IMAGING
Post-treatment assessment is typically performed with either contrast-enhanced CT or MRI, though contrast-enhanced US may also be used. On B-mode US, the treated area in parenchymal organs (liver, kidney, and spleen) is generally hypoechoic but can be difficult to differentiate from surrounding normal parenchyma. Through the use of contrast-enhanced US, treatment zones demonstrate lack of contrast enhancement, but patent blood vessels are visible traversing the treatment zone.28 The appearance of treatment zones by CT is well circumscribed, hypoattenuating, and without enhancement after the administration of iodinated contrast materials (Figure 3).16 By MRI, the treated areas display mild hyperintensity on T1 and T2 weighted imaging, and are similarly non-enhancing after the administration of contrast (Figure 3).24 Across all imaging modalities, treatment zones appear well circumscribed and precise, closely resembling the prescribed treatment area with minimal or no damage to surrounding tissues. For example, large blood vessels, ureters, and bile ducts are preferentially preserved due to a higher cavitation threshold. Over time, histotripsy treatment zones show rapid resorption (Figure 3) and scar formation within 1–3 months, while maintaining distinct borders from the surrounding parenchyma.8,14,16
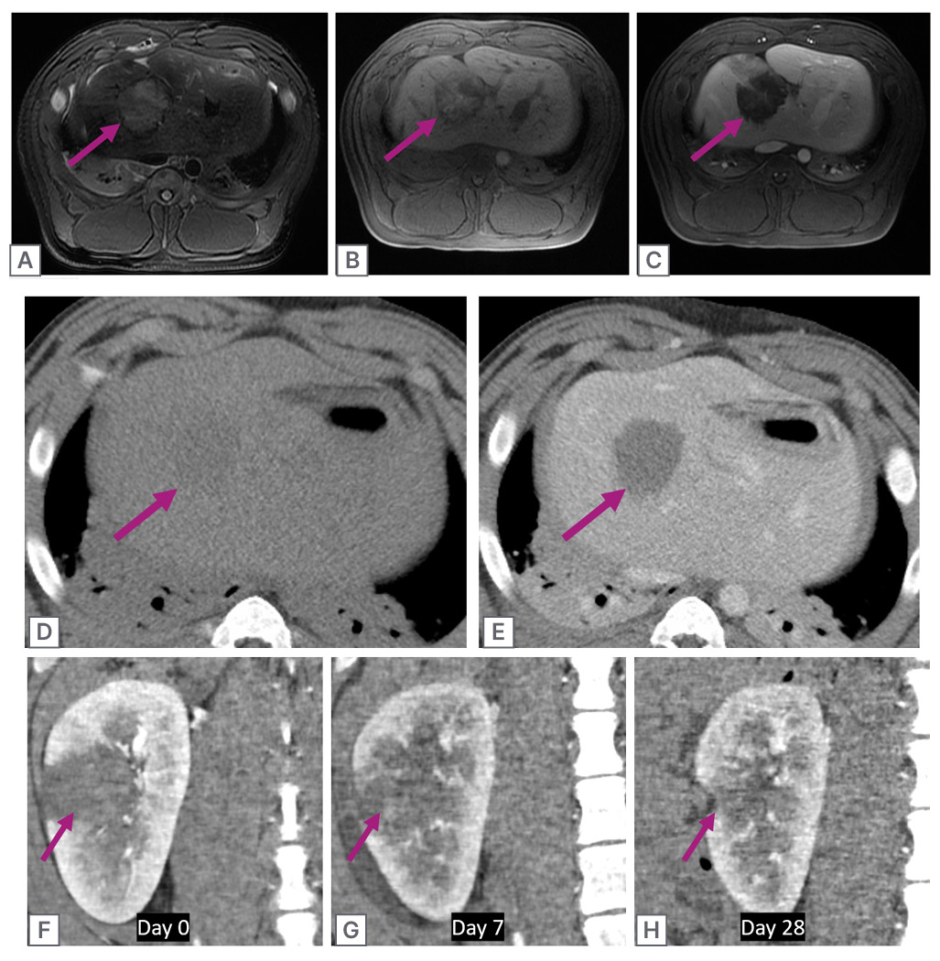
Figure 3: Post-treatment imaging.
A) Post-treatment T2 weighted MRI following histotripsy treatment in healthy swine liver shows mild hyperintensity of the treatment zone (purple arrow). B) T1 weighted MRI. C) T1 weighted MRI after IV administration of a gadolinium-based contrast agent. Note the lack of enhancement of the treatment zone.
D) Post-treatment CT shows the difficulty of treatment zone visualisation without IV contrast. E) Post-treatment CT after IV administration of iodinated contrast, which demonstrates a well-defined non-enhancing treatment zone with no collateral damage to surrounding liver. This is a typical appearance for post-histotripsy treatments.
F–H) CT imaging of the natural history of a histotripsy treatment in a healthy swine kidney over 28 days (coronal, cortico-medullary phase). Note the rapid and near-complete regression of the treatment zone that may have advantages for diagnosing residual untreated tumour.
IV: intravenous.
SUMMARY
Histotripsy is a new focused US treatment modality that destroys targeted tissue through cavitation. US can be used for targeting, cavitation monitoring, and post-treatment assessment due to a co-axially mounted diagnostic transducer contained within the therapeutic transducer. CBCT is being actively explored for histotripsy targeting due to the large open bore, which can contain the therapeutic transducer and coupling water bath, and the ability to visualise structures that may be invisible to diagnostic US. Histotripsy treatment zones are well visualized by CT and MRI and are circumscribed, precise, do not enhance after the administration of intravenous contrast materials, and rapidly resorb, leaving behind small areas of necrosis and scarring.