INTRODUCTION
Conditions impairing bladder function in children and adults require an augmentation cystoplasty and other reconstructive procedures upon the failure of conservative therapy. Despite providing functional improvement, these procedures are associated with significant long-term complications such as bladder stone formation, recurring urinary tract infections, and malignancies, resulting in a strong clinical need for alternative therapies for these reconstructive procedures.1-3
Within the last years, various natural,4,5 synthetic,6 and hybrid7 scaffolds for bladder engineering have been developed. However, improved bladder regeneration and the replacement of defective bladder tissue with functional equivalents have not been achieved yet and remain one of the major challenges in the field of bladder tissue engineering. Hence, the objective of this study is developing functional smooth muscle tissue for detrusor muscle replacement and improving the native detrusor muscle regeneration in a damaged bladder wall.
MATERIAL AND METHODS
Primary rat bladder smooth muscle cells (rSMCs) and adipose-derived stem cells (rADSCs) have been isolated and expanded, and rADSCs were differentiated into rSMC-like cells (pADSCs) using an established protocol. After flow cytometric characterisation, the rSMCs and pADSCs were mixed in ratios of 1:1, 1:2, and 1:3 (rSMCs:rADSCs) and grown for ≤3 weeks in culture. In addition to the two-dimensional (2D) co-culture, compressed collagen (CC) hydrogel scaffolds containing these cell compositions were fabricated. Cells grown in the 2D and three-dimensional (3D) microenvironments were evaluated and compared concerning their morphology, SMC-marker expression, viability, proliferation, and functionality (contractility). Furthermore, the effect of direct, cell-to-cell co-culture, and indirect co-culture using Transwell® (Sigma-Aldrich, St. Louis, Missouri, USA) inserts was assessed by viability test and in/on-cell western blot analyses.
RESULTS
After defining the optimal cell culture conditions the co-cultures showed high viability and proliferative capacity in the CC scaffolds, which led to the development of interconnected cell layers and microtissue formation in the hydrogel after 1 week. A significantly increased cellular proliferation was observed in all directly co-cultured specimens compared to the monocultures. Indirect co-culture led to an increased pADSC-survival and a cell ratiodependent improvement of rSMC-proliferation. The overall pADSC-proliferation was also increased, yet unaffected by the cell ratio. Over 8 days of growth the 1:1 ratio exhibited the steadiest proliferation compared with the 1:2 and 1:3 ratios. A strong expression of the rSMC-specific markers α-smooth muscle actin, calponin, MyH11, and smoothelin was detected after 1 week, becoming rather pronounced after 2 weeks. The expression of all rSMC-specific markers over all cell ratios reached nearly the native rSMC-marker expression after 2 weeks of co-culture and the contractility did not reach significance among each other and compared to the rSMC monoculture after 48 hours. See Figure 1.
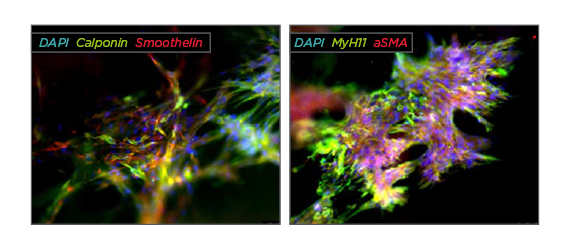
Figure 1: Immunofluorescence staining of rSMC:pADSC co-culture in a 1:1 ratio after 2 weeks of growth in a compressed collagen scaffold.
CONCLUSION
In summary, our results showed an improved cell proliferation and survival of SMC-pADSC co-cultures compared to mono-cultures of each cell type in 2D cell culture experiments. Further 3D-CC experiments confirmed that in fact a co-culture of these cell types proves beneficial for the formation of smooth muscle tissue. Thus, the combination of rSMCs and pADSCs with CC may help to engineer functional detrusor muscle tissue by overcoming current limits of tissue engineering, namely poor primary cell survival, proliferation, phenotype instability, and tissue function.