Abstract
Most public health statistics outline the rapidly exploding burden of Type 2 diabetes mellitus as a chronic endemic disease related to sedentary lifestyle and obesity. Tremendous efforts and resources are being invested in finding new medical treatments and alternative therapies through cell-based replacement strategies among other methods. Several types of cells continue to be under active research, including autologous islet cells, allogeneic cadaveric islet cells, embryonic and induced pluripotent stem cells, bone marrow-derived hematopoietic and mononuclear cells, and mesenchymal stem cells of different sources. The objective of this review is to bring the reader up to speed on the efforts being spent in this field with a clear and critical approach to the difficult and sometimes futile methodology undermining the results obtained.
INTRODUCTION
Over the past decade, the potential of stem cells has been explored with amazement, myths, and many more questions than answers. The dream was that we might be able to repair almost any malfunction inflicting our bodies. The promise of taking off-the-shelf cells or even body parts, making better targeted pharmaceutical agents, and even defying death, all fired our imagination. Along that evolving field, the regulatory authorities proceeded shyly. The biggest hurdle was the challenge to the scientific method, which served us well for >200 years; however, questions have arisen regarding its adaptability to new clinical research requirements. As a result, data that were felt to provide overly weak evidence, lack the proper statistical weight, and be poorly designed and executed, inundated the scientific arena and split the scientific community for and against stem cell research.1,2
Diabetes has become a major endemic problem around the world with a very expensive price tag. Its incidence is on the rise, fuelled by our digital lifestyle and the growing endemic of obesity. The International Diabetes Federation (IDF) estimates that in 2015, there were 415 million diabetic adults aged 20–79 years worldwide, including 193 million undiagnosed subjects. Another 318 million adults are estimated to have pre-diabetes or impaired glucose tolerance and insulin resistance. The cost of diabetes care is estimated at $673 billion per year. The American Diabetes Association (ADA) estimates that roughly 9.3% of the American population is diabetic with about 2–3-times as many possibly having pre-diabetes.3,4
The pancreatic islets of Langerhans are tiny clusters of cells scattered throughout the pancreas consisting of several groups of specialised cells, including insulin-producing beta cells. The pathology of Type 2 diabetes mellitus (T2DM) combines an inflammatory–autoimmune microenvironment affecting the insulin-production units, which become unresponsive to demand. More importantly, a dysfunction of the insulin receptors ensues, leading to peripheral insulin resistance. Another component of the pathobiology is widespread microvascular disease, which leads to many of the clinical manifestations and complications accompanying T2DM, including limb ischaemia and ulcers, retinal damage, impotence, neuropathies, nephropathies, and cardiovascular and cerebral-vascular diseases.5-8
Cellular therapy for diabetes has adapted different protocols and methods due to uncertainty regarding the best cells to use, optimal cell number, route of administration, and schedule. Many cells remain under investigation, including embryonic reprogrammed cells, pancreatic islet-derived replacement cells, reprogrammed induced pluripotent stem cells (IPC), mesenchymal stem cells (MSC), bone marrow mononuclear cells (BM-MNC), and others.
PRIMARY ISLET CELL REPLACEMENT
Allo and autotransplantation of islet cells were two of the earliest methods used to replace damaged beta cells. The allotransplantation, consisting mainly of infusion of cadaveric islet cells into the hepatic artery, is used mostly for Type I diabetes mellitus, but major drawbacks existed as patients had to be on lifelong immune suppression to prevent immune rejection. Allografting was mostly carried out between 1999 and 2013 and documented by the Collaborative Islet Transplant Registry (CITR). One thousand and eleven allogeneic islet transplants were carried out by 2013, mostly in North America. Autologous islet transplant is performed following total pancreatectomy in cases of severe, chronic pancreatitis. The islets are infused back into the hepatic artery or directly into muscle to reimplant and regain function in a new, less aggressive microenvironment.9
INDUCED ISLET BETA CELLS
The lack of sufficient sources of islet cells, need for immune-suppression when performing allotransplants, and the high mortality and morbidities associated with islet cell transplantation led to the need for new sources to replace the damaged beta cells in diabetes.
Different cells, including embryonic, MSC, IPC, hepatocytes, fibroblasts, and neurons were shown to be transformable into beta islet cells using sequential expression of one or several differentiation genes leading ultimately to the beta-cell phenotypes capable of releasing insulin on demand.10 Using the IPC technology on autologous cells, several factors and media were shown to promote this transformation. Oct4, Nanog, and Pdx1 are differentiation factors expressed early in pancreatic embryogenesis. Other factors, including Sox17, FoxA2, Pdx1, Nkx6.1, and Ngn3, may be used to induce transformation into functional beta cells. Factors like Pax4 have been shown to induce alpha cell transformation into beta cells in vitro. Pdx1, Ngn3, and MafA were shown to transform acinar cells into beta cells.11-13
Ramiya et al.14 isolated pancreatic ductal epithelial cells from prediabetic, adult, and non-obese mice and allowed them to proliferate in long-term cultures. The group then induced the production of functional islets that responded in vitro to a glucose challenge, and reversed insulin-dependent diabetes in mice.14 Xu et al.15 used MSC in vitro to produce insulin-secreting beta-like cells using recombinant adenoviruses carrying Pdx1 or Pdx1 along with Pax4. Mihara et al.16 showed that induced expression of Pdx1 and Nkx6.1 factors could result in insulin producing cells, on demand, using a three-dimensional suspension bioreactor system and stage-specific growth factors. Another approach used was the addition of bone morphogenetic protein inhibitors and protein kinase C activators to produce beta cells from embryonic stem cells.17,18
Another innovative approach used specific micro-RNA (miRNA) to overexpress in umbilical cord-derived MSC. miR-375 and miR-26a have been shown to have the capability to induce differentiation into insulin-producing cells.19 Chandra et al.20 generated IPC from murine adipose tissue-derived stem cells, and restored glycaemic control within 2 weeks in mice.
Both the bone marrow and the Warton’s jelly stem cells were shown to form islet-like clusters in a medium containing nicotinamide, activin, hepatocyte growth factor, exendin-4, and pentagastrin.21 Neuron-conditioned medium was also shown to have the ability to differentiate IPC into normal functioning beta cells in vivo using a stepwise culturing approach.22
While all these advances bring a lot of hope to the field, only preclinical data are available and the clinical exploitation of each of these techniques remains a few years and many trials away before the clinical safety, efficacy, and usefulness become evident.
HAEMATOPOETIC AND BONE MARROW CELLS
There are about a dozen studies using peripheral and bone marrow derived CD34+ haematopoietic cells claiming effective diabetes control in human studies. The injection of the prepared cells into the arteries feeding the pancreas was used in most studies. The side effects were mild, including abdominal pain, nausea, and discomfort. The efficacy was again shown in several studies using glycated haemoglobin (HbA1c), C-peptide levels, and the need for fewer medications and insulin.23 (Table 1).
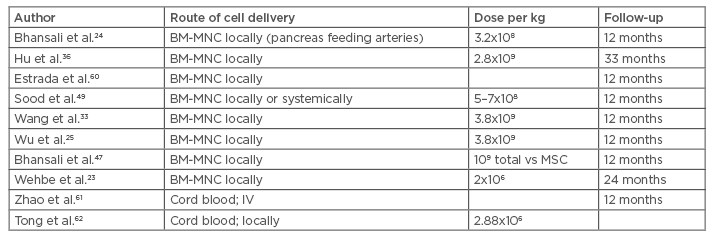
Table 1: Clinical trials using bone marrow hematopoietic and mononuclear cells to treat Type 2 diabetes mellitus.
BM-MNC: bone marrow mononuclear cells; IV: intravenous; MSC: mesenchymal stem cells.
Autologous bone marrow contains hematopoietic stem cells, a mixture of mononuclear cells, a few mesenchymal cells, and other cells. Peripheral blood stem cells are mainly selected by their CD34 antigen positivity. Different preparations of the hematopoietic cells have been claimed to be effective in correcting hyperglycaemia, improvement of endogenous insulin production, and diminishing or eliminating the need for insulin and other diabetes controlling treatments.23-26 There is no evidence of in vivo BM-MNC differentiation into pancreatic beta cells;27 there are other potential mechanisms to explain this improvement, including neovascularisation, endothelial repair, inflammatory environment modulation, and endogenous stem cell stimulation through paracrine mediators.28,29 The hematopoietic cells were shown to stimulate angiogenesis and vascularisation in ischaemic areas;30 their interaction with the microenvironment was also shown to stimulate the tissue’s own endogenous stem cells to replace the injured cells resulting in repair.29 By virtue of these processes, an anti-fibrosis effect was often observed.
The bone marrow can be separated by centrifugation with or without Ficoll-Paque density gradient into different sections. The bone marrow stem cells move to the injured areas where they secrete different growth factors and interact with the microenvironment.30,31 No significant adverse events or safety issues have been reported to hinder the administration of these cells, except for procedure-related pain, minor bleeding, or haematomas.23-33 Wang et al.33 used autologous bone marrow to treat 31 patients with stem cell infusion into the major arteries feeding the pancreas. The HbA1c dropped by >1.5% within 30 days and the C-peptide increased at the 3-month follow-up mark. All patients were reported to have had a significant reduction of their anti-diabetic medications.25
BM-MNC were used by Bhansali et al.24 in a prospective, randomised, placebo-controlled trial designed to treat 11 patients. Altogether, 9 of the 11 patients (82%) achieved 50% reduction of the insulin requirements and 10 (91%) achieved a HbA1c <7% in the intervention group. The C-peptide elevation is the surrogate used to demonstrate activation of the endogenous insulin production.34 Wu et al.25 reported treating 80 patients with BM-MNC with or without hyperbaric oxygen. The bone marrow was shown to help control the diabetes, partially or completely in some cases, with no effect of the hyperbaric therapy.35 Hu et al.36 treated 118 patients with BM-MNC or insulin only. The BM-MNC were infused into the pancreas feeding arteries. The HbA1c and C-peptide levels improved significantly.36
Finally, two recent meta-analysis of published trials concluded that both BM-MNC and peripheral blood mononuclear cell infusion may result in improvement of the HbA1C, fasting plasma glucose, C-peptide levels, and endogenous insulin production at 12 months in the majority of treated patients. These two publications compiled several studies differing in terms of the number of cells used and routes of infusions, lacking the statistical significance and the proper randomisation design. Nevertheless, these data were exploited to support powerful conclusions and claims of safety and effectiveness.11,37,38 Again, the duration of response varied between 6 months and 2 years as assessed by the HbA1C.
UNDIFFERENTIATED MESENCHYMAL STEM CELLS
MSC are pluripotent progenitor cells with proven regenerative, angiogenic, anti-fibrosis, immunomodulatory, and growth-stimulating properties. The MSC can be isolated from different tissues in culture, including the placenta, bone marrow, adipose tissue, and fetal cord, among others. In T2DM, the MSC may achieve more than one goal, including endothelial blood vessel repair, balancing of the immune environment, stimulation of the endogenous stem cells, and ultimately islet cell repair and replacement. The MSC can be allogeneic or autologous and have to be sequentially cultured in specially defined conditions exploiting the property of attachment to the culture dishes.39,40
Even the conditioned medium where the MSC are cultured were found to have similar capacity and properties to regulate glucose in diabetic mice, indicating a major paracrine contribution.41 The MSC create a microenvironment that promotes the endogenous cells to proliferate and regain function.40 Some paracrine factors have been identified, like the vascular endothelial growth factor alpha, platelet-derived growth factor, angiopoietin-1, and insulin-like growth factor.41
The known immune regulatory and immune privileged properties of MSC with a low expression of Class II major histocompatibility complex and co-stimulatory molecules allow them to suppress the proliferation of T and B lymphocytes and modulate their immune responses, including antibody formation, cytotoxicity of T and natural killer cells, B cell maturation by promotion of the T cell tolerance, and induction of the proliferation of regulatory T cell populations.42,43 The sum of these processes may lead to the sparing of the pancreatic cells from the ongoing destruction. MSC have been claimed to induce autophagy as well and by this mechanism promote cellular repair and wound healing.43,44 Several studies provided evidence that MSC may help alleviate the insulin resistance encountered in T2DM. The reversal or attenuation of the insulin resistance may be mediated by the cytokines released and possibly their effects on the macrophages, but the exact mechanisms remain far from understood. No significant acute allergic or immunologic events have been reported but occasional mild nausea, vomiting, headaches, abdominal pain at the puncture site, haemorrhage, or haematoma may occur (Table 2).45-52
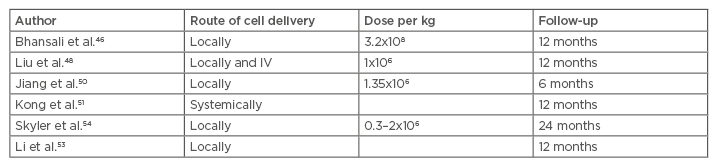
Table 2: Clinical trials using mesenchymal stem cells to treat Type 2 diabetes mellitus.
IV: intravenous.
The fate of the infused cells was explored by Sood et al.49 who gave radio-labelled fluorodeoxyglucose bone marrow MSC (BM-MSC) to mice intravenously or into the superior pancreatico-duodenal and splenic arteries. Positron emission tomography tracking showed that the MSC given through the intravenous route homed to the lungs before spreading through the systemic circulation. The locally injected cells partially migrated to the pancreas. The HbA1c reduction and insulin requirements were proven to be superior when the cells were injected directly into the pancreas feeding arteries.49
Bhansali et al.46 infused BM-MSC to treat 10 patients and showed reduction of the insulin requirements; in addition, 3 patients were able to discontinue insulin completely. Wang et al.43 reported a reduction in the HbA1C levels as early as 1 month. The same phenomenon was also observed in two other clinical trials carried out by other researchers; HbA1C was found to diminish starting at 3 months and lasting 1 year on average.50-52 The effect of the cells was shown to persist between 9 months and 2 years.43,50-52
Multiple MSC injections were used in some trials in an attempt to prolong their effects, but no clear results have been reached on the best schedule of injection and there is no consensus on the optimal time or interval of MSC infusion in diabetic patients. What is clear from different reports is that the effect is typically limited to several months, although it may persist up to 2 years.49,51,52
Another important issue to consider is the best dose of the effector cells. While most studies used a cell number dependent on the weight, on average 106–2.6×107cells/kg are being used.38 The response has been reported to be dose-dependent in some, but not all, studies.53 The effects on hyperglycaemia were observed early on in some studies but the C-peptide followed later, peaking at 6 months. The effects, on average, lasted about 12 months following MSC implantation. Hu et al.52 reported that the normalisation of the C-peptide peaked at 1 year and declined over the following 2 years.48,50,51
Skyler et al.54 published a milestone Phase III, placebo-controlled, multicentre clinical study in July 2015 using allogeneic MSC at an escalating dose. The target HbA1c of ≤7% was achieved by 33% (5 of 15) of the subjects who received the 2.0×106 cells/kg dose and 50% of those receiving 1.0×106 cells/kg. The source of the MSC was adult allogeneic bone-marrow precursor cells. Only one dose of MSC was given intravenously with no significant side effects reported. No patients discontinued the study because of an adverse event.54
Finally, Domouky et al.55 transplanted 40 rats with BM-MSC or IPC with differentiated insulin producing cells. Both groups showed improvement of diabetic control but the IPC group displayed normal regeneration and distribution of the beta cells in the pancreas and resumption of endogenous insulin production to a more significant degree.55
DIABETIC COMPLICATIONS
The pathobiology of diabetic complications is closely related to the diabetic vasculopathies and the inflammatory microenvironment. On the clinical platform impotence, neuropathies, wound healing, renal failure, retinal damages, cardiac failure, and leg ulcers, among other complications, can make life exceedingly challenging for T2DM patients. Several trials have shown efficacy, or at least improvement, of the diabetic complications. Cao et al.56 used BM-MSC to show improved healing of diabetic foot ulcers. Packham et al.57 showed that the infusion of allogeneic mesenchymal precursor cells in patients suffering from diabetic nephropathy using a commercially available allogeneic MSC (Rexlemestrocel-L®, Mesoblast, New York City, New York, USA) is safe and tolerable for human use and may stabilise or improve diabetic nephropathy.57 Xiang et al.58 showed that rats treated with BM-MSC conditioned medium had enhanced vascular remodelling after an induced stroke. Zhang et al.59 reported restoration of vision in diabetic retinopathy in rats after intra-vitreal injection of neural stem cells originating from human umbilical cord-derived MSC. Treatment of diabetic rats prevented the decline in brain- derived neurotrophic factor levels usually caused by diabetes.59 Intralesional BM-MNC injections into skin ulcers and its surroundings have been shown to improve the limb perfusion and ulcer healing in patients with critical limb ischaemia.31
CONCLUSION AND FUTURE DIRECTIONS
There are about 50 ongoing studies on stem cell use in T2DM that are registered at www.clinicaltrials.gov. There has been a large number of small published studies that indeed do not constitute a solid scientific proof of the efficacy of different stem cells being tried. Due to most published data lacking statistical significance and a blinded randomisation scheme, we feel that it is futile to employ meta-analytical methods to draw useful conclusions. One big issue is whether cells are comparable between laboratories. In other words, we do not have enough data to assume each study is not reporting on a different cell subtype, due to missing flow cytometric identification in most studies. The introduction of pre-prepared or frozen cells, like the MSC of umbilical or bone marrow origin, by different pharmaceutical companies has proven extremely expensive at this point and definitely out of reach to the vast majority of individuals. Larger studies are needed to advance the field and understand the best way to realise its potential. We believe stem cell therapy should only be used within clinical trials at this time, until enough evaluable data becomes available. Caution has to be exerted to expand the regulatory work necessary to frame this new field and help it enhance our armamentarium against T2DM. The laboratory methods, like culture conditions and methods of cell numbering, have to be better thought of and more uniformly standardised, and the interpretation of the results should be done critically. Many biases have to be excluded by adopting better double blinded randomisation, statistical methods, and large enough sample sizes. Most researchers in the field are working through the preclinical phases of experimenting with the differentiation of different stem cells into insulin-producing beta cells while another group is exploiting the anti-inflammatory properties of the bone marrow and MSC. The future of stem cells may be heading towards restoring and executing both strategies at the same time.