Abstract
Recent National Institute for Health and Care Excellence (NICE) guidelines suggest that insulin pump therapy should be used in pregnant women with Type 1 diabetes mellitus (T1DM) who do not achieve optimal glycaemic control with multiple daily injection (MDI) therapy. Furthermore, a landmark trial has confirmed that prospective continuous glucose monitoring (CGM) may be beneficial for women using both MDI and insulin pumps during pregnancy, with positive effects on neonatal outcomes. More recently, overnight use of an artificial pancreas (AP) with a model-predictive control algorithm has been shown to improve the amount of time women spend within the overnight glucose target range (3.5–7.8 mmol/L) during pregnancy. However, preliminary studies where the AP is used day and night have shown a high degree of interindividual variability in response to the intervention, and further randomised trials are needed to understand which women are suitable candidates for CGM, insulin pump, and AP technology. It is understood that improvements in maternal glycaemic control can minimise the risk of adverse neonatal outcomes. Given the substantial improvements in glycaemic control with AP use outside of pregnancy, the recent advances in AP technology provide hope that AP systems will improve the effectiveness of continuous subcutaneous insulin infusion and CGM during pregnancy. Further research is needed to evaluate whether AP can optimise glucose control and neonatal outcomes in T1DM pregnancy. This paper will discuss emerging technologies available for the management of T1DM in pregnancy.
INTRODUCTION
Type 1 diabetes mellitus (T1DM) in pregnancy increases the risk of obstetric and neonatal complications associated with maternal hyperglycaemia.1 These widely recognised complications include higher rates of congenital anomaly, macrosomia, shoulder dystocia, stillbirth, and neonatal death.2 The primary goal for these women is to improve their glycaemic control, thereby improving maternal fetal outcomes.3
The physiological state of pregnancy is diabetogenic and therefore the additional hyperglycaemia of T1DM can have significant adverse effects.4 The decreased response to insulin observed during pregnancy has often been attributed to an increased level of progesterone, oestrogen, prolactin, human placental-lactogen, and resultant ‘increased placental-fetal glucose demands’.4,5 As gestation progresses in T1DM, hormonal and other factors cause fluctuations in insulin requirements, making insulin dosing difficult to predict.6 Further, women with T1DM are 3–5-times more likely to experience severe hypoglycaemia during early pregnancy compared with the period before conception, and some women, especially those with impaired awareness of hypoglycaemia, experience recurrent hypoglycaemia episodes throughout pregnancy.7 It is hoped that diabetes technology will miminise the burden of hypoglycaemia.
PREVALENCE AND EPIDEMIOLOGY
In the UK, approximately 700,000 women give birth annually and 2–5% of these pregnancies are complicated by diabetes.8 Of these, around 88% involve gestational diabetes, 5% involve Type 2 diabetes mellitus, and 7% involve T1DM.8 The rate of neonatal death (death of a live-born infant up to 28 days after delivery) in T1DM pregnancies across the UK is currently 8.1 in every 1,000 births, 2–5-times higher than the non-diabetic population. This remains unchanged from 2002.1 Across the UK, the only pregnancy outcome to significantly improve since 2002 is the rate of stillbirth (fetal loss after 24 weeks’ gestation), which is 2.5-times lower than the figure obtained in the 2002–2003 Confidential Enquiry into Maternal and Child Health (CEMACH) (10.7 versus 25.8 in every 1,000 births, p=0.0012).1 This decrease is believed to be largely due to changes in obstetric management, which now recommends more timely induction of labour for women with diabetes, since there have been no improvements in glycaemic control during T1DM pregnancy.9
Despite the advances in diabetes management and widespread use of insulin analogues, in the UK, only around 14.3% of women with T1DM manage to achieve adequate glycaemic control during the first trimester.1 Moreover, continuous glucose monitoring (CGM) data demonstrate that women with T1DM are spending an average of 12 hours per day in the target glucose range during early pregnancy.11
MEASUREMENT OF GLYCAEMIC CONTROL DURING PREGNANCY
Continuous Glucose Monitoring
CGM measures the level of glucose in the interstitial fluid through a subcutaneous sensor and transmits the information via radio frequency to a data display receiver or smartphone, providing real-time glucose monitoring. For masked or professional GCM, the glucose data is uploaded from a docking station and only available to patients, clinicians, and/or researchers retrospectively. CGM provides between 96 and 288 glucose measurements per day, with in-depth information on glucose fluctuations and trends over time.12 This information enables users to visually appreciate the feedback that their diet and physical activity has on their glycaemic control and can serve as an important educational resource.12
At present, there are four main types of CGM systems available: retrospective, real-time, on-demand, and implantable. Retrospective CGM continuously records information regarding glycaemic control; however, this information is not visible to the user in real-time and is instead downloaded for retrospective evaluation of glucose trends to facilitate lifestyle and pharmacological modifications.13 Given the frequency of fluctuations in glucose levels during pregnancy, prospective adjustments to insulin delivery based on retrospective glucose information will not adequately address all women’s needs. As such, the applicability and usefulness of retrospective GCM during pregnancy is limited.
Real-time CGM provides users with immediate feedback on their blood sugar levels, allowing users to see the impact of exercise, sickness, and carbohydrate intake on their glycaemic control.14 Flash glucose monitoring does not require calibration with capillary glucose testing, and uses a 14-day sensor that, when scanned, provides users with their current glucose level (thus referred to as on-demand monitoring) and a graph of glycaemia since their previous scan.15 Furthermore, the U.S. Food and Drug Administration (FDA) has recently approved the use of the implantable Eversense® CGM device (Senseonics, Germantown, Maryland, USA).16 Available in Europe and the USA, this device has a fluorescence-based sensor that is implanted subcutaneously. A transmitter worn above the sensor sends information to a mobile phone app that displays the glucose values and their trends. Compared with current CGM devices, which need to be replaced up to every 14 days, the Eversense lasts up to 3 months.16 Eversense has not yet been evaluated for use in pregnancy, but it is assumed that its accuracy during pregnancy would be comparable to outside pregnancy, as per other sensors.17
Two early trials of CGM during pregnancies complicated by T1DM suggested no benefits of CGM over traditional monitoring techniques. A 2013 study by Secher et al.18 showed no improvement in glycaemic control or neonatal outcomes when real-time CGM was used intermittently (on average five times) during pregnancy. The subsequent ‘Glucomums’ randomised controlled trial,19 comparing retrospective CGM with conventional treatment, also failed to show any improvement in glycaemic control or neonatal outcomes with CGM use. In both studies, both real-time and reterospective CGM were used intermittently. Outside pregnancy, it has been established that real-time CGM needs to be worn >70% of the time for a significant improvement to be seen.20
More recently, the results from the large, robust, CONCEPTT study21 were published. This multicentre, open-label, randomised controlled trial allocated 215 pregnant women and a further 110 who were planning pregnancy to CGM or capillary glucose monitoring alone. The results demonstrated a modest improvement in HbA1c with CGM use (-0.19%, 95% confidence interval [CI]: -0.34–-0.03; p=0.0207) and more time-in-target range (68% versus 61%; p=0.0034). This translated into significant clinical improvements in the CGM group, with a lower incidence of large for gestational age (LGA) infants (odds ratio [OR]: 0.51, 95% CI: 0.28–0.90; p=0.0210) and fewer neonatal intensive care unit (NICU) admissions over 24 hours (0.48; 0.26–0.96; p=0.0157).21
The NICE guidelines (Section 1.3.18, revised in 2015)3 recommend real-time CGM use for women with problematic severe hypoglycaemia, unstable blood glucose levels (to minimise variability), and to gain information about variability in blood glucose levels. However, only 16% of T1DM women achieve the NICE glycaemic control target of HbA1c <48 mMol/Mol (6.5%) in early pregnancy and almost all have unstable glucose levels.1 As of July 2018, NICE announced that, based on findings from the CONCEPTT trial, it is planning a partial update of its guidelines for CGM use in T1DM pregnancy.22
Currently, CGM is not subsidised for pregnant women on the NHS. The costs of real-time monitoring over 28 weeks (from 10–38 weeks gestation) can be up to £2,500.23 However, this needs to be offset against the cost of not subsidising the technology. In the CONCEPTT study, the rate of NICU admissions >24 hours was 27% in CGM and 43% in the control group (OR: 0.48, CI: 0.26–0.86; p=0.0157).21 The mean cost of hospitalising a neonate in a level 3 NICU is >£17,861.24 This benefit alone, notwithstanding the other clinical benefits, provides a compelling basis for performing cost-benefit analyses for GCM during T1DM pregnancies.
In addition to using CGM as a tool for guiding insulin dosage, there is a move to use GCM data as a measure of the adequacy of glycaemic control. During pregnancy, there are physiological changes that render HbA1c a less effective measure of glycaemic control. For example, red blood cells during pregnancy have an increased affinity for glucose.25,26 The CONCEPTT trial confirmed the clinical relevance of time-in-target as an appropriate outcome measure during pregnancy. A 7% increase in time-in-target range during pregnancy was associated with a significant (approximately 50%) reduction in neonatal complications.21
Continuous Subcutaneous Insulin Infusion (Insulin Pumps)
Outside of pregnancy, it has been widely established that continuous subcutaneous insulin infusion (CSII) is safe and is associated with improved glycaemic control.27,28 However, the benefits of the technology during pregnancy are less well established.
CSII is thought to be a more ‘physiologic’ method of insulin delivery because it is closer than other forms of insulin delivery to the pattern of insulin secretion from the pancreas.29 When purchased privately, CSII can cost between £2,000 and £3,000 in the UK.30 These pumps can be programmed to have different basal rates at different times of the day according to the need of the individual user, which is a key advantage over multiple daily injection (MDI) therapy.31 Notably, in a pre-specified secondary analysis of CONCEPTT data, it was found that MDI users had a larger decrease in HbA1c and were more likely than CSII users to achieve target HbA1c.32 The offspring of MDI users had fewer NICU admissions and reduced levels of gestational hypertension.32 These findings should be interpreted with caution due to baseline differences in maternal smoking and hypertension, but they suggest that CSII implementation during pregnancy may be suboptimal.
A Cochrane Review33 of four studies performed in 2007 that compared CSII with MDI during pregnancy concluded that there were no differences in neonatal outcomes or glycaemic control with use of the technology. However, the first study did not include any information about the randomisation process and the second study recruited a skewed sample population of highly educated, highly motivated participants.33 The possibility of bias in these studies can therefore not be excluded. Furthermore, the sample sizes were small and lacked statistical power to detect between group differences in obstetric and neonatal outcomes.
It has been postulated that the improvement in glycaemic control when using CSII is due to the ability of the technology to facilitate more frequent fine-tuning of the dosages of insulin.27 Yet, due to conflicting evidence, CSII is not routinely recommended during pregnancy. The NICE guidelines now reflect this uncertainty by recommending its use in pregnancy when adequate glycaemic control is not obtained with MDI use.3
Sensor Augmented Pump Therapy
Sensor augmented pump therapy (SAP) is a combination of CGM and CSII therapy that allows for close monitoring of glucose levels and tighter adjustments of insulin dosing.34 The CGM and insulin pump communicate in real-time to store and transmit information regarding glycaemic control. This information can be uploaded to the internet for use by people with diabetes and their clinicians.34
The potential benefits of SAP were demonstrated in the STAR 335 trial that showed that the addition of CGM to CSII in non-pregnant adults resulted in decreased HbA1c levels and less time spent in a hypoglycaemic state. In CONCEPTT, participants using CSII had a significant improvement in the percentage of time-in-target with the addition of CGM, suggesting that CGM improves the effectiveness both of MDI and of CSII.21 A pilot study in pregnant women with T1DM compared SAP with CGM and demonstrated a lower HbA1c level in the SAP group (6.52% versus 6.82%; p<0.05).36
Closed-Loop Insulin Delivery Device (Artificial Pancreas)
The closed-loop insulin delivery device, or artificial pancreas (AP), is an automated insulin delivery system comprised of a CGM, a CSII, and an algorithm that amalgamates real-time information from the CGM to adjust insulin delivery (Figure 1).37,38
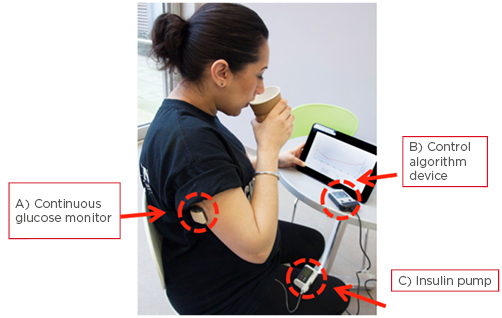
Figure 1: Study participant using the closed-loop insulin device.
Components of a closed-loop system: A) continuous glucose monitor (sensor, transmitter, and receiver); B) a control algorithm device; and C) an insulin pump.
Adapted from Stewart.38
Outside of pregnancy, the AP has been shown to be effective at improving glycaemic control and quality of life in children and adults without increasing rates of hypoglycaemia, when compared to conventional treatment or SAP.37,39,40 A recent meta-analysis of 24 randomised control trials (N=585) demonstrated that, outside of pregnancy, the AP improved time-in-target by 3 hours compared with stand-alone pump therapy (target range: 3.9–10.0 mmol/L).41 AP also reduced the amount of time spent in a hypoglycaemic state by almost 50% (from approximately 5.0% to 2.5%).41 In 2016, the FDA approved the use of Minimed™ 670G (Medtronic, Dublin, Ireland), which uses a proportional-integrative-derivative (PID) control algorithm, as the first commercially available AP.42
Minimed 670G is a hybrid automated system that replaces basal insulin doses, but the device still requires the user to self-administer prandial boluses via the insulin pump. The control algorithm that has been assessed during pregnancy is the model-predictive control (MPC) algorithm.17,43 This algorithm “predicts future glucose excursions based on patients’ glycaemic responses to insulin and meals”.44 There are a number of different algorithms that have been trialed in the AP. In their 2017 meta-analysis of 24 studies, Weisman et al.41 demonstrated that the improvement in time-in-target with the AP compared with CSII is approximately 12.6%, regardless of the algorithm used. PID was associated with a slightly lower time-in-target compared to fuzzy logic and MPC algorithms, although this was not statistically significant.41
The first two Closed-Loop In Pregnancy (CLIP) studies, CLIP 01 and 02, were randomised, crossover pilot studies that demonstrated the safety and efficacy of implementing a MPC algorithm in pregnancy.17,43 The subsequent CLIP 03 study was an open-label, randomised crossover study comparing overnight closed-loop therapy with SAP in 16 pregnant women with T1DM.45 The women completed 4 weeks of closed-loop therapy (intervention) and 4 weeks SAP (control) in random orders, followed by a continuation phase, in which the closed-loop system was used day and night until discharge from hospital after delivery.45
The study demonstrated a significant improvement in the percentage of time overnight spent in the target blood glucose range of 3.5–7.8 mmol/L with use of the AP (74.7% versus 59.5%; absolute difference: 15.2% points; 95% CI: 6.1–24.2; p=0.002). The mean overnight glucose level was also lower with AP use compared with the control (6.6 versus 7.4 mmol/L; p=0.009), and AP use was not associated with an increase in hypoglycaemia frequency.45 There was no statistically significant improvement in HbA1c,45 although this is unsurprising given the relatively short study period and the limitations of using HbA1c measurements in pregnancy.46 The system was able to cope well with the physiologic challenges of pregnancy, including antenatal steroid administration, labour, and anaesthetics.45
Although the observed improvements in glycaemic control were promising, there was a higher than expected number of adverse obstetric and neonatal outcomes in this study. Thirteen of the fetuses were LGA, 12 required a NICU admission, 5 women had pre-eclampsia, and 15 women delivered by caesarean section.45 However, the study population were high-risk and included a number of women with a poor obstetric history. Further analyses of neonatal outcomes are not possible because the randomised intervention lasted only 4 weeks and the crossover trial was not designed to assess neonatal outcomes.
The most recent study, CLIP 04,47 had an almost identical study design to CLIP 03; however, the participants used closed-loop day and night during the intervention phase, rather than only overnight as in CLIP 03. Participants in CLIP 04 showed no significant difference in the proportion of time spent in the target day-and-night regions with AP use (62.3% versus 60.1%, p=0.47), although fewer hypoglycaemic episodes occurred with AP use (time spent <3.5 mmol/L, 1.6% versus 2.7%, p=0.02).47
The rates of adverse neonatal outcomes in CLIP 04 were more in line with clinical expectations than in CLIP 03 (2/16 women had pre-eclampsia, 7/16 infants were LGA, and 11/16 were admitted to the NICU).47 However, it is noteworthy that a number of factors may have resulted in the varied outcomes of CLIP 03 and 04. Firstly, CLIP 04 examined AP use during the day as well as overnight. It is far more challenging to achieve ideal glycaemic control during the daytime, when meals, snacks, exercise, and premeal bolusing are factors.47 Moreover, in CLIP 04, during the SAP control phase, participants spent 60% of the time within the pregnancy target range of 3.9–7.8 mmol/L (during day-and-night). This ‘control’ rate is substantially higher than in normal control groups outside of pregnancy, and was comparable to or higher than the degree of control achieved previously in other studies of AP.37,39 This minimises the potential for further improvement with the AP.
In CLIP 04, there was a high degree of inter-individual variability with some women demonstrating improvements in glycaemic control with the intervention and others having no improvement with the intervention.47 Notably, a broad population range were recruited in CLIP 04 with the women coming from a range of educational backgrounds and socio-economic statuses and having varying levels of baseline glycaemic control. Furthermore, >80% of participants were sensor naïve.47 In a sample size of 16 patients, these factors can significantly influence the results of the studies.
There were a number of limitations to CLIP 03 and 04. The relatively short 4-week study duration may not be sufficient to train device-naïve participants. Moreover, the cross-over study design may not have been ideal for participants with variability in their lifestyle. However, overall, these four proof-of-concept studies investigating day-and-night closed-loop insulin delivery demonstrated that the technology may be able to safely achieve acceptable glycaemic control during pregnancy.
Recently, a prespecified secondary analysis of the data from CLIP 03 and 04 was performed.48 It aimed to identify the baseline characteristics of the study participants that could predict a positive response to the AP. Women with lower baseline HbA1c had a more significant biomedical improvement (p=0.014) than women with a higher baseline HbA1c.48 Although the exact reason for this is unclear, researchers postulated that the same factors that precipitated optimal preconception glycaemic control, such as high levels of health motivation and health literacy, facilitated the optimal implementation of closed-loop. Age, BMI, duration of diabetes, location of diabetes treatment, and previous experience with CSII had no effect on the treatment effect.48
Women were willing to bear the burdens and limitations of the device, including frequent malfunctions and physical bulkiness, if they perceived that it improved their biomedical control and quality of life.48 Reported short comings of the technology included frequent alarm malfunctions, slow connections between the CGM and the CSII, and the cumbersome nature of carrying around a device. In contrast, the same women noted improved control, a strong sense of mental freedom resulting from the lesser requirement to calculate insulin dosages, improved sleep, and a reduced sense of hypo and hyperglycaemia-related anxiety with the AP.48
In 2019, a parallel-arm, randomised controlled trial of 124 women across 10–12 NHS antenatal diabetes centres in England, Scotland, and Northern Ireland will be conducted. The open-label AiDAPT trial will assess the clinical efficacy of the AP on glycaemic control in pregnant women with T1DM. This trial will use the Dexcom® G6 CGM (Dexcom, San Diego, California, USA), which does not require users to perform fingerprick calibrations.49
CONCLUSION
Despite new technologies and recommendations about how best to manage T1DM in pregnancy, obstetric and neonatal outcomes remain suboptimal. CGM, insulin pumps, and the AP have shown promise in the management of the disease; however, the evidence regarding the use of these technologies is rapidly evolving as the devices develop and it is challenging for healthcare professionals to remain up-to-date with this ever-changing landscape.
With the advent of each new promising diabetes technology, there has been hope for a product that will achieve optimal glycaemic targets with minimal effort and disruption to daily life. However, the benefit of CSII has been modest at a population level and further research is required before the efficacy and cost-effectiveness of SAP can be robustly concluded.38 Given the complexity of diabetes management, it is likely that no single technology will be able to cure the condition. Therefore, any technology that manages to safely and efficaciously improve glycaemic control and quality of life of pregnant women with T1DM is worth considering. At present, considering the data from CONCEPTT, the authors suggest implementing GCM into routine care of pregnant women with T1DM. The addition of CSII should be considered on a case-by-case basis, with clinicians considering baseline glycaemic control, patient preference, and affordability.
The AP represents an exciting frontier in the management of T1DM in pregnancy. In studies to date, AP has shown an ability to cope with the physiologic challenges of pregnancy and may lead to improvements in diabetic outcomes such as an increased time spent in target. The technology was well received by participants and almost all of the women in the AP trials elected to continue using it after the crossover phase of the study, rather than returning to their previous therapy.45,47 However, further evidence from randomised controlled trials is needed to understand whether AP can help to bridge the gap between clinicians expectations of tight glucose control during pregnancy and the reality of day-to-day glucose excursions experienced by patients.