Key Points
1. Tuberculosis has a mortality rate of 50% if treatment is not provided, but with timely detection and interventions, 85% of people can be cured.
2. India, being a resource-poor country, has one of the highest burdens of tuberculosis in the world, with an incidence of 210 out of 100,000 people in 2021.
3. This article discusses the commonly used diagnostic methods in nations with limited resources, outlining the main challenges, whilst advocating for potential resolutions.
INTRODUCTION
Tuberculosis (TB), rightly referred to as an ancient disease, has affected humans for thousands of years, the first drafted reference of which came from India and China around 3,300 and 2,300 years ago, respectively.1 TB, caused by a bacillus called Mycobacterium tuberculosis, is a deadly infectious disease that is transmitted through aerosol droplets, and is estimated to have infected one-quarter of the global population. It has a mortality rate of 50% if treatment is not provided; however, with timely detection and interventions, which include currently recommended anti-TB drugs, 85% of people can be cured.2 India, being a resource-poor country, has one of the highest burdens of TB in the world, with an incidence of 210/100,000 in 2021, according to the World Health Organization (WHO) Global TB report of 2022.2
The global impact of TB is marked, and it is estimated that nearly one-third of the human population is afflicted by it. In 2021, the WHO recorded a surge in the incidence of TB and drug-resistant TB, which marks the first upturn in approximately two decades. This upsurge is concurrent with an increase in mortality rates. It is worth noting that only eight countries account for approximately two-thirds of all cases, one of which is India.2
During the timeframe spanning 2016–2020, the WHO employed three distinct global lists of high-burden countries in relation to TB; TB co-infection with HIV; and multidrug-resistant TB (MDR-TB), respectively. Notably, India features in all the three lists. Therefore, India is contending not just with the incidence of TB, but also with MDR-TB and TB/HIV.
The primary objective of this article is to conduct an overview of the challenges encountered in the diagnosis of TB within a setting that possesses limited resources, whilst advocating for potential resolutions. Furthermore, a comprehensive analysis of the prevailing practices for the laboratory diagnosis of TB is provided.
DIAGNOSTIC MODALITIES OF TUBERCULOSIS
The diagnosis of TB is complex because, although the WHO has established guidelines for the disease’s diagnosis and treatment, each country has employed an algorithm-specific approach. Here, the commonly used diagnostic methods in nations with limited resources are discussed.
Microscopy
In low-resource settings, the diagnosis of TB is still based on sputum smear microscopy, where samples are labelled ‘smear positive’ or ‘smear negative’, based on the results of Ziehl–Neelsen (ZN) stain.3 Only around 50% of all active TB cases are identified. Nonetheless, it is the most practical, simple, cheap, and accurate method of diagnosing the disease in TB-prevalent countries.4 Two samples (spot and early morning) are required for diagnosis under India’s current National TB Elimination Programme (NTEP), as a result of which, the patient must make multiple visits to healthcare facilities in order to get screened for TB by sputum microscopy.5 The rise in diagnostic expenditures has caused a significant number of patients to discontinue follow-up care amidst ongoing diagnostic procedures. The new WHO criteria for smear positivity facilitates the use of a single smear for follow-up purposes.4 Relying solely on sputum microscopy for diagnosing TB may pose a public health risk, as smear negative cases, which account for 17%, are known to transmit the disease.6 Routine microscopy cannot be used to detect non-tuberculous mycobacteria, or utilised as a follow-up diagnostic test because of its incapability to differentiate between live and dead bacilli. Despite numerous drawbacks, such as the requirement of a large number of bacilli for detection, quality of material, and expertise of the laboratory worker, it is a convenient tool in the rudimentary labs that are standard in underdeveloped countries.3,7,8
Light-Emitting Diode Fluorescent Microscopy
Light-emitting diode fluorescent microscopy (FM) outperforms ZN microscopy in many aspects. The approach is more resilient; easily operated; has better battery life, with no ultraviolet (UV) light generation; and is low-maintenance, allowing areas of the world where there are a lack of resources to benefit from FM.3,9 The effectiveness and sensitivity of microscopy results of a smear are enhanced by using FM, the disadvantage being the fading of the stain over time. These microscopes provide a much broader view of the smear, allowing for a quicker analysis of the specimen (up to four-times faster), and easier bacilli counting.4,10,11 The NTEP, which received WHO sanction in 2009, has brought in the use of light-emitting diode FM to substitute the ZN technique in increased workload-designated microscopy centres across India.8
Culture
Culture, including both conventional egg-based (Löwenstein–Jensen) and agar-based methods (Difco™ Middlebrook 7H10/11 Agar, Avantor Inc, Radnor, Pennsylvania, USA), allows for the detection of antimicrobial resistance, including new mutations. Growth in a Löwenstein–Jensen medium takes 4–8 weeks, with an additional 4 weeks for drug sensitivity using the conventional proportion approach. Consequently, standard culture methods require an average of 70 days to diagnose a case of MDR-TB.12
Few developing nations are able to conduct high-quality drug-sensitivity tests (DST) on second-line medications, and even fewer on first-line drugs. Most countries reserve TB culture for treatment failure or patients who are drug-resistant, and sending samples to distant labs might cause delays in the processing of samples and, ultimately, in test findings. Biosafety cabinets are a prerequisite for performing both conventional and automated culture methods. While culture remains the gold standard method to diagnose TB with a 99.99% specificity, as summarised in Table 1, there is always a risk of contamination.3
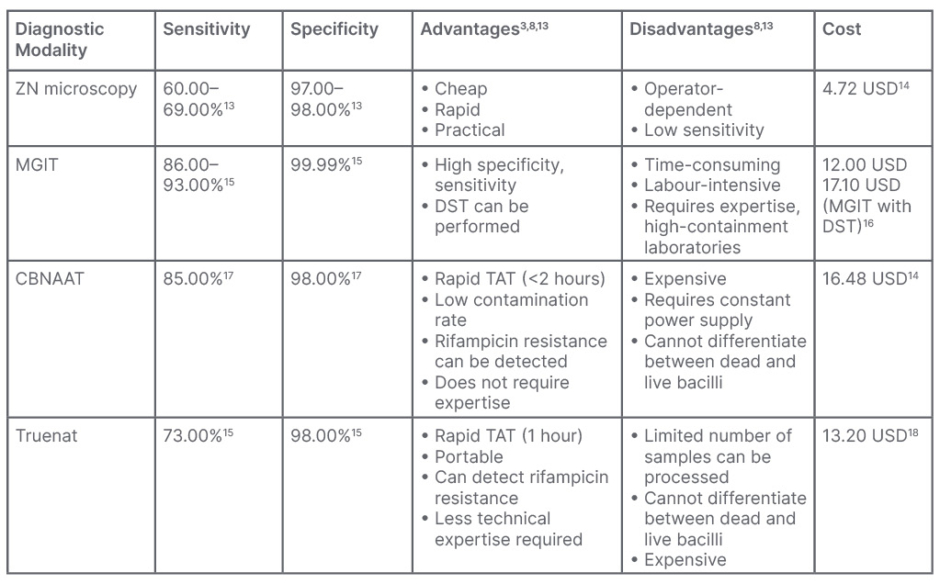
Table 1: Costs of commonly used diagnostic tools for tuberculosis, with associated advantages
and disadvantages.
CBNAAT: cartridge-based nucleic acid amplification test; DST: drug-sensitivity tests; MGIT: MGIT 960 System; BACTEC™ MGIT™ (Becton, Dickinson and Company, Franklin Lakes, New Jersey, USA); Truenat: Molbio Diagnostics, Goa, India; ZN: Ziehl–Neelsen.
Liquid Culture Media
NTEP has introduced the WHO-endorsed mycobacterial growth indicator tube (MGIT 960 System; BACTEC™ MGIT™, Becton, Dickinson and Company, Franklin Lakes, New Jersey, USA), a liquid culture using Middlebrook 7H9 broth that evaluates O2 consumption by fluorescence for detection of M. tuberculosis and DST, and is compatible with all types of clinical specimens except blood.4,8
When the O2 in the culture is used by the bacteria, it becomes luminescent when exposed to UV light. Similar to microbiological methods, DST uses two culture samples: one with and one devoid of the drug (a growth control), and is known as one percent proportion testing sensitivity method.19 The growth of tubercle bacilli and the fluorescence will be suppressed if the test medicine is effective against them. With the manual system, a technician uses a portable UV lamp-containing device to visually identify fluorescence. Automatic systems may handle up to 960 different cultures simultaneously.4
When inoculating decontaminated samples, an antibiotic cocktail of polymyxin B, amphotericin B, nalidixic acid, trimethoprim, and azlocillin (BBL™ MGIT™ PANTA, Becton, Dickinson and Company) is added to the culture tube containing oleic acid, albumin, dextrose, catalase (BBL™ MGIT™ OADC, Becton, Dickinson and Company), and 7 mL of Middlebrook 7H9 broth base. The degree of fluorescence, measured by a fluorescent sensor, is proportional to the amount of O2 used by the microorganisms in the inoculated samples which, in turn, correlates with the number of bacteria present, and is considered positive. The test is deemed superior to the standard egg-based solid culture approach due to its low turnaround time (TAT) and higher mycobacterial recovery; however, it has a higher contamination rate (6–8%) than the conventional method (4–5%). TAT for M. tuberculosis complex recovery is 10–14 days, with an additional 6–10 days on average for DST results.8,20,21 This method can detect mycobacteria other than tuberculosis, and DST can be carried out for both first- and second-line anti-TB medicines.8,22 Each culture grown using these methods must be tested using an immunochromatographic assay for the detection of M. tuberculosis complex or ZN staining.8
Genotypic Method
Cartridge based nucleic acid amplification test
Clinical specimens can be tested for M. tuberculosis and rifampicin (RIF) resistance using the cartridge-based nucleic acid amplification test (CBNAAT), a semi-quantitative, nested, real-time PCR. In 2010, this approach was approved by the WHO for the detection of pulmonary and extrapulmonary TB, as well as for the detection of TB in children.3 However, in case of extrapulmonary TB, NTEP does not advocate the use of urine, stool, and blood. Using ultrasensitive hemi-nested PCR (by amplifying 81-base pairs hot spot region of rpoB gene of M. tuberculosis) and molecular beacon technology, the GeneXpert® (Cepheid®, Sunnyvale, California, USA) MTB/RIF assay may detect M. tuberculosis complex (MTBC) and related RIF susceptibility status directly from clinical samples.8 Current NTEP guidelines recommend it for drug resistant-TB (DR-TB) diagnosis in presumptive DR-TB, and for first TB diagnosis in high-risk groups, such as children, individuals with extrapulmonary TB, and those with HIV.
Sample type has an effect on how well tests perform with data from patients with extrapulmonary TB. More effective detection of M. tuberculosis has led to the development of a new version, Xpert® MTB/RIF Ultra (Cepheid®), which was released recently. The Ultra cartridge has enhanced performance, now detecting 16 bacilli per mL sputum sample, by including two new molecular targets for M. tuberculosis detection. Paucibacillary TB, such as those caused by HIV-TB co-infection, paediatric TB, extrapulmonary TB, and acid-fast bacilli smear-negative TB, benefit from this improved sensitivity.8,23 Specifically for the diagnosis of presumptive DR-TB cases, NTEP has updated the diagnostic algorithm to permit the use of CBNAAT instead of microscopy in a phased approach.23 It has a faster TAT and a much lower contamination rate, but requires a constant power supply for it to function. Furthermore, its incapability to distinguish between live and dead bacilli makes this diagnostic modality unsuitable for follow-up cases.3
Truenat MTB test
The WHO has approved a portable chip-based nucleic acid test for the detection of M. tuberculosis.24 If the initial test for M. tuberculosis in a sample is positive, the extracted DNA can be re-tested for rifampicin resistance with the Truenat MTB-Rif Dx chip (Molbio Diagnostics, Goa, India). A TruLab® microprinter (Durham, North Carolina, USA) can be used to print the results. However, the analyser includes built-in connectivity, so the results can be sent over a wireless connection, Bluetooth, or a SIM card. There are three stages to the test: liquefaction and cell destruction (sample preparation); DNA extraction and purification; and amplification and fluorescence probe-based detection.25 Truenat assays for detecting M. tuberculosis and RIF resistance were shown to be just as accurate as the Xpert MTB/RIF assay (Table 1).8
OTHER MODALITIES FOR DETECTION OF TUBERCULOSIS
Antigen Detection
MPT64, a TB-specific antigen released by MTBC during development, is detected by strip speciation test in positive cultures in 15 minutes. Most laboratories in India use this antigen detection for quick identification of MTBC in liquid culture.3
Lipoarabinomannan
The detection of lipoarabinomannan, a glycolipid, takes around 4–6 hours and 20 minutes by ELISA (Creative Diagnostics®, Shirley, New York, USA) and dipstick, respectively.3,4,7 The WHO only recommends lipoarabinomannan detection in urine for TB diagnosis in patients with HIV with TB symptoms and a CD4 of count <100 cells/L.
Line Probe Assay
After DNA extraction and PCR amplification, this strip test can detect TB DNA and genetic alterations linked with treatment resistance in smear positive sputum specimens or culture isolates. Different species of Mycobacterium can be distinguished using this hybridisation experiment. Although the TAT is 4–6 hours in theory, the full process can take up to 72 hours in practice. When done on smear-positive and culture-isolate samples, its sensitivity and specificity are both high. NTEP at intermediate reference laboratories in India has implemented line probe assay, which is also recommended by WHO for MDR-TB.3
Loop-Mediated Isothermal Amplification for Detection of Mycobacterium tuberculosis (TB-LAMP)
TB-LAMP, a commercial version of LAMP (Eiken Chemical Company, Tokyo, Japan), is a nucleic acid amplification method. Eiken’s Loopamp MTBC Detection Kit (TB-LAMP) targets MTBC gyrB and insertion sequences regions, detects the presence of amplified product in less than 1 hour, and can be read with unaided eye or under UV light. The WHO suggests employing TB-LAMP in place of microscopy for detection in symptomatic people with signs of TB. In adults with pulmonary TB symptoms, it can be used as a follow-up to microscopy, especially for sputum smear-negative specimens.26
Whole Genome Sequencing
Over the past 10 years, whole-genome sequencing of M. tuberculosis has revolutionised tuberculosis research.27 Single nucleotide polymorphisms, identified by genome sequencing of the bacteria, can be used to predict susceptibility to first-line drugs,28 distinguish strains and lineages,29 or study outbreaks and transmission events.30-32 The lengthy and laborious procedure of growing M. tuberculosis for DNA extraction has limited the use of this method in the routine diagnosis and surveillance of tuberculosis. Most high-burden countries cannot afford these procedures, and direct whole-genome sequencing is less sensitive than culturing.32
Detection of Extrapulmonary Tuberculosis
Extrapulmonary specimens can be divided into two groups: sterile body fluids like spinal, pleural, pericardial, synovial, peritoneal, blood, bone marrow, tissues (lymph node, tissue biopsies), and fine needle aspirates; and specimens contaminated by normal flora: gastric lavage, bronchial washings, urine, pus, and stool (in individuals who are HIV-positive and infants with disseminated TB).33
Due to the paucibacillary nature, extrapulmonary TB specimens have a poor sensitivity of about 10%. Although culture is the gold standard for detection of TB, the result depends upon the type of extrapulmonary TB specimen being processed.34-36 Xpert® Ultra has been used in extrapulmonary specimens in much research. The sensitivity ranges from 47.6–84.2% for pleural fluid to 50.0–100.0% for lymph nodes and cerebrospinal fluid, and has shown high performance in paediatric and TB/HIV groups.37
A useful biomarker, adenosine deaminase in peritoneal fluid, has been seen as a good tool for screening and diagnosing peritoneal TB in India, with a pooled sensitivity of 93% and specificity of 95%, according to a systematic review.38 However, Xpert® MTB/RIF is much more rapid in terms of diagnosis, with a high specificity, and can replace conventional microscopy, culture, and histopathology for testing specimens like lymph nodes and other tissues from patients suspected of extrapulmonary TB (conditional recommendation, very low-quality evidence).37,39
CHALLENGES IN THE LABORATORY DIAGNOSIS OF TUBERCULOSIS
In high-incidence countries like India, the laboratory diagnosis of TB is fraught with multiple obstacles. In addition to the lack of resources, the sheer volume of patient inflow attributes to the challenges faced, amongst many others. The heavy reliance on ZN microscopy for the diagnosis of TB is a widely acknowledged fact, despite the numerous limitations. Due to the lack of access to sophisticated diagnostic tools and adequate healthcare facilities, diagnosis is often delayed, causing a greater burden on the patient and the healthcare system. This issue highlights the need for expanded access to advanced medical technologies and point-of-care diagnostic tools in poor-resource settings, to enhance the timely detection and management of TB. However, it is understood that implementation of such a solution requires a protracted timeline and is complex, thereby demanding a nuanced methodology. Here, the various problems that institutions in developing countries face and potential solutions are elaborated.
Inadequate Infrastructure
A crucial element of a good laboratory lies in its infrastructure. A laboratory infrastructure includes not just a well-designed building that is work-flow centric, but prioritises safety and adaptability as well. The National Accreditation Board for Testing and Calibration Laboratories (NABL), which is in alignment with International Standard (ISO 15189; 2012) mentions in detail the environmental conditions applicable in laboratories. They include the following requirements: sufficient space conducive to smooth operation, a pleasant environment, and measures that prevent cross-contamination; effective segregation of activities that are not compatible, for example, the autoclave for sterile articles and for decontamination should not be kept together; enough room for patient reception, sample collection, workstations, equipment, and the safe storage of potentially hazardous materials, such as volatile and inflammable reagents and biohazardous substances; provision of continuous and reliable power backup, electrical outlets, and adequate lighting; a seamless support system for all computers, peripherals, equipment, and communication devices to ensure uninterrupted service; and separation of the sample processing site from the area designated for testing, whenever feasible.40
TB, being a highly infectious disease, mandates the use of a biosafety laboratory that adheres to the set guidelines. The majority of laboratories in developing countries do not have the funding or the means to obtain recognition as per national or international standards, which is the main cause behind the following commonly encountered obstacles. Outdated constructions of laboratories, characterised by antiquated design, with limited potential for adaptability or expansion, constitute most of the institutions. This proves to be challenging not only for the employees, but also creates difficulties for the patients’ orientation, the bulk of whom belong to impoverished segment of the population. Another matter identified pertains to patients originating from geographically remote locations who, as a result of the obscure and indistinct infrastructure, experience significant delays in their treatment journey. This is commonly associated with misplacement of specimens or the need for repeated sample collection. There is a clear deficit of suitable biosafety-level laboratories, and the prevalent issue of accumulation of expired reagents and dysfunctional equipment, which results in unnecessary occupancy of space. Disrupted Internet connectivity is frequently faced, and hinders the processing of the Laboratory Information Management System (LIMS), which is vital for report dissemination and patient information.
Insufficient Manpower: Health Workers and Laboratory Staff
The vital role of laboratories in domestic healthcare systems is gaining recognition on a global scale. Despite the challenges faced by resource-limited nations, one major issue they face is the significant shortage of skilled professionals in laboratory settings.41
The paucity of human resources, coupled with a high volume of samples, restrict the potential of laboratory diagnosis of TB in numerous ways. Diagnostic modalities, such as ZN staining microscopy, are heavily operator-dependent, and a high sample load may adversely impact the accuracy of the report outcome. The issue of inadequate workforce in countries grappling with TB endemicity, poverty, and overpopulation is far from being a straightforward predicament. Rather, it is accompanied by various factors, like absence of secure working conditions, appropriate equipment, personal protective gear, training programmes, and regular medical evaluation.
During the period of 2011–2015, a survey was conducted in the Asia Pacific region on diagnostics laboratories. The survey indicated that India had a significantly high ratio of specialised personnel to laboratory when compared to developing and developed nations. It also states that the levels of computerisation and equipment are relatively lower in the Indian laboratories.42
In order to combat the shortage of human resources in financially-challenged nations, it is necessary to explore solutions beyond merely increasing funding.
Supply Chain Management
A diverse range of diagnostic modalities are available for detecting TB, as outlined within this review. Nonetheless, poor maintenance and upkeep of the equipment, and delayed repair or supply, consistently impede operational efficiency (Figure 1). Some examples that can be cited would be: the delay in repair of modules of important molecular diagnostic tools like CBNAAT and Truenat by weeks and even months, which in turn results in a substantial reduction in testing capacity; interrupted supply of reagents and kits essential for the consistent functioning of laboratory equipment; and delayed restoration of malfunctioning biological safety cabinets, which is crucial to the secure operation of the laboratory and its personnel (Figure 1).
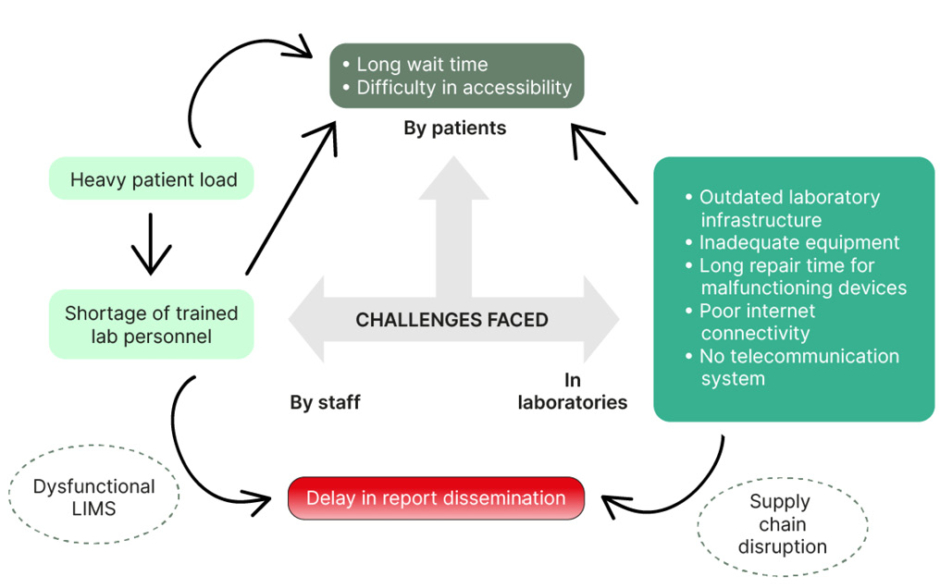
Figure 1: Schematic diagram of major challenges encountered in the laboratory diagnosis of TB in low-resource settings.
LIMS: Laboratory Information Management System; TB: tuberculosis.
OVERCOMING CHALLENGES IN TUBERCULOSIS DIAGNOSIS
Technology-Enabled Services
A well-functioning LIMS should be made a standardised practice among all physicians and data operators. The benefits of a smoothly operating LIMS are several, including easier management, tracking, and profiling of samples; effortless generation of reports owing to real-time, advanced, and integrated dashboards; minimised paperwork; increased productivity; and efficient utilisation of time for health workers and patients. Also useful would be the use of mandatory hospital-generated patient identification, and the installation of computers and a telecommunication system within hospital wards and laboratory facilities.
Laboratory Infrastructure and Management
Establishment of safe laboratory designs and environment that include well-ventilated and sufficiently spaced laboratories with minimal risk of cross-contamination and a straightforward and consistent setup, wherein the areas for sample collection and processing are well demarcated; the provision of adequate and functional biosafety cabinets and protective equipment for the laboratory staff; and strict implementation of standard operating procedures in each laboratory.
Human Resources
More recruitment of skilled manpower, and training should be provided to the senior staff regarding the management of human resources, workload, and workplace organisation, forecasting and ordering of supplies, budgeting, and oversight of quality assurance.40 In this day and age of science and technology, the extension of training can be achieved through portals like telemedicine, videoconferencing, and webinars. A crucial, yet often overlooked, measure that would significantly improve the long-term outcome would be to ensure periodic medical check-ups of laboratory personnel, given their extensive exposure to contagious illnesses.
Logistics
The appointment of a competent supply manager to oversee procurement and inventory operations within all laboratory settings should be made mandatory, which could include strict monitoring and maintenance of laboratory stock with documentation, and regular reports of the logistics data. Effective communication and collaboration is necessary among the pharmacy, supply management, and laboratory units.40
The task of creating the optimal conditions and circumstances in a country with limited resources is far from achievable in a short timeframe. However, we can continue to pursue endeavours towards enhancing our performance with the mentioned strategies, and broader goals involving the policy-makers and the government (Figure 2).41
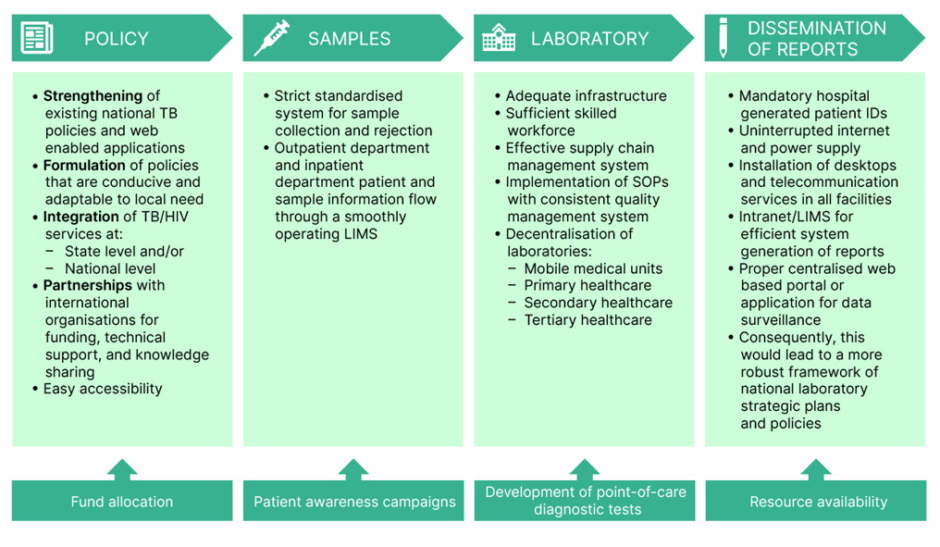
Figure 2: Schematic diagram of strategies to overcome challenges in the diagnosis of tuberculosis in resource-limited countries.
ID: identification; LIMS: Laboratory Information Management System; SOP: standard operating procedures; TB: tuberculosis.
CONCLUSION
The Indian government has implemented several measures to mitigate the impact of TB on patients and society. These measures comprise subsidised or cost-free treatment options, financial and nutritional assistance programmes, incentive-based initiatives, and thorough monitoring practices aimed at providing effective follow-up care for patients with TB.
However, being a resource-poor country, there are enormous challenges, like insufficient funding, high population density, increasing cases of MDR-TB and TB with HIV, and general lack of awareness. To overcome these challenges would require a lot of time and collective effort from various sectors, such as the government, the healthcare industry, and the general population. Given that the COVID-19 outbreak has impeded efforts to eliminate TB, there is a heightened sense of urgency to initiate measures to terminate this lethal and contagious disease using the assets and capabilities available presently. In this article, the authors have outlined some of the most common obstacles that are encountered during the laboratory diagnosis of TB, as illustrated in Figure 1. Although point-of-care diagnostic technologies are currently in the research and development phase, their availability remains distant, even after their completion. Therefore, certain strategies that can be employed to address this complex predicament have been elucidated, with emphasis on technology-enabled services. It is crucial to acknowledge that in order to progress, it will always be fundamental for healthcare providers to thoroughly evaluate the correlation between laboratory findings and clinical observations, so that accurate diagnosis and informed decisions can be made regarding treatment.