Abstract
Years after coronary artery bypass graft surgery, plaque formation or graft degeneration is a major concern. Saphenous vein grafts (SVG) are vulnerable to degeneration and occlusion, leading to poorer long-term disease because of atherosclerotic degeneration. The main mechanism responsible for SVG failure is neointimal hyperplasia and the occluded SVG is treated with percutaneous coronary intervention, mostly with the use of additional protection devices. Graft intervention for the diffuse degeneration of SVG can be performed with the use of suitable hardware without the distal protection device being required.
The authors herein report the case of a 63-year-old female who presented with degenerated SVG to the left anterior descending artery with anastomotic stenosis, 6 years after coronary artery bypass graft surgery. She was successfully treated with three ultra-thin sirolimus-eluting stents in SVG to the left anterior descending artery, without the use of any embolic protection device.
Learning Objectives
- Coronary intervention for the diffuse degeneration of the SVG can be contemplated when there is a ‘no-option’ clinical situation.
- Intervention of this unusual form of degeneration of venous graft has not previously been mentioned in the literature.
- Diffusely degenerated venous graft intervention requires the same approaches as chronic total occlusion intervention, such as the use of Gaia-2 (Asahi Intecc, Aichi, Japan) and the support of the microcatheter, multiple balloons for sequential predilatation, and longer length stents.
INTRODUCTION
Treatment of degenerated saphenous vein grafts (SVG) has been challenging, even in the era of escalating advancements in technology. SVG are more vulnerable to high-grade stenosis and occlusion, secondary to venous graft degeneration (VGD), as compared to native coronary arteries, such that within 10 years post coronary artery bypass graft (CABG) surgery only about 60% of SVG remain patent. VGD differs from coronary artery disease, which involves the native coronaries in morphology, as it comprises foam cells, cholesterol crystals, blood elements, and necrotic debris, and less fibrocollagenous tissue and calcification, with softer, friable, and larger plaque. However, uniquely, there can be diffuse degeneration processes that can include the entire length of the SVG, including anastomotic location. In these cases, the process of pathophysiology primarily involves the increased neointimal hyperplasia and extensive atherosclerosis, which contributes to diffuse long segment VGD and has been correlated with plaque embolisation and the occurrence of no-reflow.
All these characteristics lead to challenging interventional procedures in SVG.1 In addition, patients undergoing SVG percutaneous coronary intervention (PCI) are found to be at elevated procedural risk and in-hospital mortality relative to patients undergoing PCI of native coronary arteries. Herein, the authors present the case of a female with a diffuse, long segment of VGD with chronic total occlusion (CTO) of the anastomotic site to the left anterior descending artery (LAD). She was successfully treated in the SVG-LAD vessel with three ultra-thin sirolimus-eluting stents (SES), without using any embolic protective device (EPD).
CASE PRESENTATION
A 63-year-female presented with complaints of shortness of breath on exertion, New York Heart association (NYHA) Class III in the previous week, and bilateral pedal oedema for the past 3 days. Six years ago, she had undergone CABG with SVG grafts to her LAD and left circumflex (LCX) arteries at another hospital. Ten years previously, she had also undergone PCI to the mid-segment of the LAD using bare-metal stent. She had a known history of hypertension and bronchial asthma.
Upon examination at admission, her pulse rate was 80 bpm and her blood pressure was 120/80 mmHg. An electrocardiograph showed 2 mm downsloping ST-segment depressions in leads I, aVL, and V1-4, suggestive of antero-lateral wall ischaemia. Echocardiography demonstrated mild left ventricular dysfunction with regional motion abnormalities of the anterior wall, distal interventricular septum, and apex, and moderate mitral regurgitation (jet area: 8 cm2). Biochemical and haematological reports were normal, including cardiac enzymes. A clinical diagnosis of unstable angina was made. After therapeutic stabilisation using dual antiplatelet agents ticagrelor and aspirin, nitrates, β-blockers, and high-dose statins, angiography of the native coronary arteries showed severe stenosis of the distal left main coronary artery with multiple bridging collaterals across the in-stent occlusion of the ostial-proximal segment of the LAD (Figure 1A and 1B). The LCX was nondominant, with proximal mild disease, and was followed by total occlusion of the major obtuse marginal branch. The right coronary artery (RCA) was the dominant artery, with 50% stenosis of the distal segment with retrograde collaterals that could visualise the distal segment of the LAD (Figure 1C and 1D). The angiography of the SVG revealed streaky, thinned out, diffusely degenerated SVG to LAD, with sluggish flow and stasis of contrast at the site of anastomosis. The LAD was caused by occlusion and there was no visualisation of the distal segment of LAD beyond it (Figure 1E and 1F). The SVG to LCX was patent, with some features of VGD. The patient was advised to undergo PCI to treat the SVG graft lesion, as intervention of the native LAD–CTO (Japanese-CTO score: 2) was thought to be more complex.
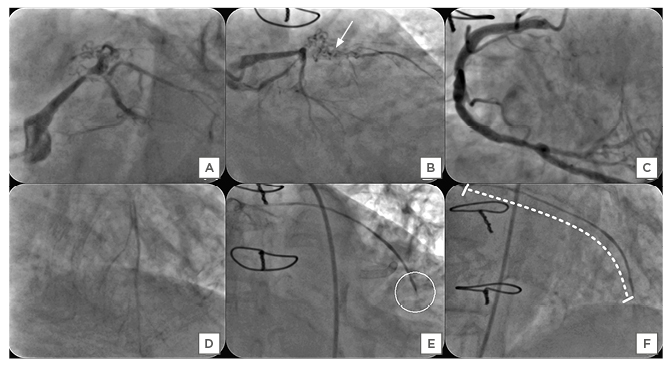
Figure 1: A–F) Angiography of the native coronary arteries in the left anterior oblique caudal (Panel A) and the right anterior oblique caudal (Panel B), showing critical stenosis of the distal segment of the left main coronary artery with ostio-proximal in-stent chronic total occlusion (CTO) of the left anterior descending artery (LAD) with multiple bridging collaterals (solid white arrow), leading to thrombolysis in myocardial infarction II flow into its distal bed (Japanese-CTO score: 2). The left circumflex artery had CTO of the obtuse marginal branch with patent saphenous vein graft supplying its territory (not shown). The right coronary angiography in the anterior-posterior cranial view demonstrated nonobstructive plaques (Panel C) with retrograde inadequate filling of LAD up to its mid segment through collaterals (Panel D). Angiography of saphenous vein graft to the left anterior descending artery in the right anterior oblique caudal, and cranial views showing diffusely degenerated graft with the slow, streaky flow and its occlusion (white circle, Panel E) at the anastomosis with mid segment of the left anterior descending artery. The venous graft degeneration involved the entire length, and the calculated saphenous vein graft degeneration score was 100% (dashed curved white line, Panel F).
After administration of 100 units/kg of unfractionated heparin, the SVG graft was cannulated with a 6 Fr Amplatz™ Left (AL)-2 (Boston Scientific, Marlborough, Massachusetts, USA) guide catheter through the right femoral approach. At the very first attempt, the SVG-LAD graft lesion was successfully crossed using a 0.014-inch Gaia-2 (Asahi Intecc, Aichi, Japan) guidewire with the support of a FineCross® microcatheter (Terumo Corporation, Tokyo, Japan) and a wire tip was placed in the distal LAD (Figure 2A).
The LAD lesion was predilated with 1.2×8 mm and 2.0×12 mm Tazuna® balloons (Terumo Corporation, Tokyo, Japan) at 12 atm for 15 seconds (Figure 2B).
The 0.014-inch Gaia-2 guidewire was exchanged for a Runthrough® guidewire (Terumo Corporation, Tokyo, Japan) (Figure 2B). Initially the Distal SVG-LAD graft lesion was stented with 2.25×44 mm Supralimus Grace (Sahajanand Medical Technologies Pvt. Ltd., Surat, India) SES, deployed at 12 atm for 20 seconds (Figure 2C). Subsequently, proximal SVG-LAD graft lesion was stented with 2.5×48 mmm SES, deployed at 12 atm for 20 seconds (Figure 2D and 2E). Another overlapping 2.5×20 mm SES was placed using StentBoost subtract visualisation software (Koninklijke Philips N.V, Amsterdam, the Netherlands) in the mid segment of the SVG-LAD graft (Figure 2F). StentBoost subtract image-guided segmental post-dilation of underexpanded portions of the deployed stents was done with 2.5×15 mm noncompliant balloons at nominal pressure of 10–12 atm. A post-procedural angiogram demonstrated no residual stenosis/dissection, with brisk thrombolysis in myocardial infarction (TIMI) III flow in the SVG-LAD graft, filling the antegrade limb of LAD beyond its mid segment, as well as multiple septal and diagonal branches (Figure 2G). Echocardiography demonstrated improvement in mitral valve regurgitation (jet area: 5.0 cm2) and left ventricular function.
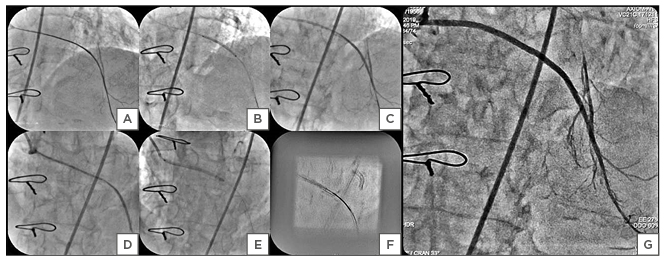
Figure 2: A–G) The percutaneous coronary intervention of the diffusely degenerated saphenous vein graft (SVG) to the left anterior descending artery (LAD). A Gaia-2 (Asahi Intecc, Aichi, Japan) wire tip was placed in the distal LAD with support of the FineCross® microcatheter (Terumo Corporation, Tokyo, Japan) (Panel A). The lesion was predilated with Tazuna® balloons (Terumo Corporation, Tokyo, Japan) of 1.2×8.0 mm and 2.0×12.0 mm at 12 atm for 15 seconds (Panel B) after the Gaia-2 wire was exchanged with the Runthrough® guidewire (Terumo Corporation, Tokyo, Japan). The distal SVG-LAD graft deployed with 2.25×44.0 mm Supralimus Grace (Sahajanand Medical Technologies Pvt. Ltd., Surat, India) at 12 atm for 20 seconds (Panel C). The proximal SVG-LAD graft deployed with 2.5×48.0 mm Supralimus Grace at 12 atm for 20 seconds (Panel D, E). Stent boost image of Supralimus Grace (2.5×20.0 mm) in the mid SVG-LAD graft, overlapping proximal and distal stents (Panel F). Post-percutaneous coronary intervention final angiography showing thrombolysis in myocardial infarction III flow in the saphenous vein graft-left anterior descending artery graft, with filling of the mid and distal segments of the left anterior descending artery and its branches (Panel G).
The patient was discharged in a haemodynamically stable condition. She was still in a favourable condition, without any related complaints, at the 9-month follow-up post PCI procedure.
DISCUSSION
With increasing numbers of CABG surgeries being performed worldwide, incidence of VGD has also been amplified, especially after >5 years post-CABG.
This has, in turn, resulted in an increase of complex SVG interventions, made more complex because SVG anastomosis to a native vessel is a predisposition to vessel occlusion, leading to development of a CTO, as in this case. However, PCI in SVG interventions are more difficult than native coronary arteries and are indicated in patients who are not candidates for redo-CABG or who have only one SVG lesion, combined with the native coronary artery lesion supplying the same territory, as in this case. There was a nondiscrete diffuse degeneration spanning the entire length of the SVG. Usually interventions for diffuse VGD are not attempted and typically aggressive medical treatment is recommended in the event of complex lesion characteristics.2
AETIOPATHOLOGY OF VENOUS GRAFT DEGENERATION
Intimal hyperplasia and advanced atherosclerosis contribute over 10–20 years to a cumulative occlusion of SVG.3 In the early phase, the technique of graft handling during cardiac surgery, including high-pressure leakage tests and exposure to the arterial circulation, leads to the venous graft distention. This leads to development of an inward remodelling, characterised by intimal hyperplasia, and a reduced lumen remodelling, characterised by mild intimal hyperplasia, depending on local and systemic influences such as inflammatory factors. In the late phase, the study of human vein grafts extracted at autopsy has also shown that coronary vein grafts undergo rapid development of atherosclerotic lesions. Lesions in coronary vein graft have a more dispersed and diffuse appearance than native lesions. Furthermore, these vein grafts have a very high propensity to rupture and occlude because of thrombosis. This may lead to focal or diffuse degeneration of the SVG. Angiographically, there can be either singular or multiple focal stenotic lesions, with or without areas of ectasia, which are more common compared to diffuse narrowing, as seen in this case.3,4
Complexities of Saphenous Vein Graft-Venous Graft Degeneration Interventions
Because of the difference in morphology and physiology of SVG grafts, proper selection of stents and EPD with the right application of good procedural techniques are required to deal with complexities, in order to reduce complications.
Firstly, the recommendation for the PCI of SVG interventions should be based primarily on patient symptoms and documentation of myocardial ischaemia in the territory supplied by the SVG. Secondly, suitable catheter selection for angiography can also reduce complications and procedural time. In the present case, upon selection of the appropriate catheter, the authors were able to cross the lesion at first attempt. Thirdly, imaging techniques such as intravascular ultrasound (IVUS) are used to assess the extent of SVG degeneration as positive remodelling on IVUS is a strong predictor of no-reflow post-intervention. In addition, the relevance of IVUS in SVG interventions is addressed below.
Role of Intravascular Ultrasound in Saphenous Vein Graft Intervention
IVUS has not been sufficiently studied in prospective SVG intervention studies to endorse intervention based on IVUS findings alone.5 Nevertheless, grey-scale IVUS has drawbacks when it comes to distinguishing between fibrous plaques and fatty plaques. This technique is less sensitive in detecting small pieces of fatty plaque, and is likely to miss them.6 Virtual histology-IVUS is used to provide quantitative assessment of both plaque composition and morphological characteristics, but there is presently no research investigating the correlation between virtual histology IVUS-identified atherosclerotic plaque components and patient outcomes in patients undergoing PCI of SVG.
IVUS can be used for the proper sizing and positioning of the stent, which is important to ensure long-term durability and patency. One study has examined the idea of undersized stenting to decrease distal embolism.7 The effects of SVG intervention using drug-eluting stents (DES) were evaluated by Hong et al.7 in three groups, using ratio of the stent diameter to the average IVUS reference lumen diameter (Group I: <0.89 mm; Group II: 0.9–1.0 mm; Group III: >1.0 mm). The elevation of cardiac enzymes more than three-times the normal was 6%, 9%, and 19%, respectively, among the three groups, without further increases in clinical adverse events. The reduction of distal embolism and periprocedural myocardial infarction with undersized stenting has to be balanced against the possible risk of increased long-term in-stent restenosis (ISR).5 Considering all these odds, the authors did not advocate the use of IVUS as there was diffuse degeneration of the graft, secondary to neointimal hyperplasia.
Selection and Technique of Implantation of Stents for Venous Graft Degeneration
The authors opted for the implantation of the undersized, latest generation DES. The latest generation DES inherently had good deliverability and crossability that posed to be of additional help towards successful graft intervention in this case. Inflation at high pressure is discouraged to minimise a ‘cheese grater’ effect, which may increase the risk of distal embolisation. It is also necessary to reduce post-stent manipulation to reduce embolisation.8
Hence, in this case, only under expanded segments of the deployed stents, detected with the help of the StentBoost subtraction imaging technique, were post-dilated, sufficiently sized, noncomplaint balloons recommended at nominal pressure.
Correlation Degree of Venous Graft Degeneration Failure-Saphenous Vein Graft Degeneration
Measurements of angiographic variables like angiographic evidence of thrombus, lesion length, vessel angulation>450°, and novel parameters like the SVG degeneration score, enabled estimation of plaque volume, which was associated with 30-day major adverse cardiac events (MACE). This included composite of death, myocardial infarction, and target vessel revascularisation.9 In this case, as the whole length of the SVG was degenerated, the calculated SVG degeneration score was 100%. The estimated plaque volume could not be calculated as there was no normal segment of the SVG to measure the reference vessel diameter.
Data relating to sex differences is controversial. Some suggest male patients are prone to poorer outcomes, while some studies quote vice versa. However, in many studies, female patients have been inclined towards considerably higher rates of vascular complications and post-procedural acute renal failure compared to male patients.5
Guideline-Based Therapies Intervention and Role of Embolic Protective Device in Saphenous Vein Graft Interventions
The current guidelines include a Class I recommendation for the use of EPD for the intervention of SVG. While Coolong et al.10 identified 30-day MACE predictors in patients treated with EPD, their effectiveness in routine practice is questionable; several recent studies, including the National Cardiovascular Data Registry (NCDR) CathPCI Registry, showed no advantage of routine EPD use. Comparing all-cause mortality, MACE, myocardial infarction, or target vessel revascularisation in SVG intervention with and without EPD, in a meta-analysis by Paul et al.11 which involved 52,893 patients, indicated no obvious advantage in the regular use of EPD in contemporary real-world practice during SVG interventions. Sometimes interventions of SVG are technically demanding because of an inability to track hardware because there could be inadequate proximal support and long diffuse lesions without a sufficient landing zone that precludes the use of EPD.12
The intervention of long segment, diffuse VGD with occlusion at site of anastomosis is a Class III recommendation, according to the current recommendations of the guidelines, but there
are numerous publications in the literature regarding effective SVG–CTO interventions, as in this case. Debski et al.13 reported a case of refractory angina in a post-CABG patient who had patent left internal mammary artery to LAD, but occluded SVG to RCA, with CTO of the native RCA. Initial antegrade and retrograde approaches could not cross the native RCA-CTO. Hence, during a second attempt, they could cross SVG to RCA with CTO using retrograde approaches across the septal collaterals but could not use EPD because of an insufficient landing zone, as in this case.13 Garg et al.14 registered a success rate of 79% in their retrospective analysis of PCI for SVG-CTO. The authors suggest that SVG intervention should be attempted whenever there are technical difficulties in re-canalising the native vessel.
In view of the aforementioned statements by the literature, this case appears to be interesting as it constitutes most predictors for adverse events, i.e., acute coronary syndrome, diffuse long-segment VGD, anastomotic site occlusion, complex CTO of native coronary artery, significant ischaemic mitral regurgitation, and female sex. Despite presence of various adverse predictors, the case was dealt with successfully without use of any distal embolic devices, occurrence of any post-procedural complications, or MACE at 9-month follow-up.
FUTURE RESEARCH DIRECTIONS
Strategies to Prevent or Impede Venous Graft Degeneration
Constrictive remodelling, intimal hyperplasia development, dysfunctional atherosclerotic lesions, and local and systemic inflammation are the key causes of VGD. In addition to the reduction of cholesterol and the pleotropic effects of statins, the specific targets of the different inflammatory factors have great potential to prevent VGD in future.3 While hyperplasia in the smooth muscle cell (SMC) is a characteristic of VGD, therapies to inhibit various cell-cycle interactions may be an important method of suppressing smooth proliferation of muscle cells in VGD, and knowledge may also be learnt from previous investigations of DES. Cancer research has also identified several important therapeutic inhibitors such as verteporfin, a tyrosine kinase inhibitor.15
The proinflammatory cytokine interleukin-1β also plays various roles in atherothrombotic plaque formation by promoting monocyte and leukocyte adhesion to endothelial vascular cells (VEC), as well as vascular SMC proliferation. Anti-inflammatory therapy targeted with canakinumab resulted in a significantly lower risk of cardiovascular event recurrence than placebo in the CANTOS study; this was shown to be irrespective of the reduction in lipid levels in patients with elevated C-reactive proteins >2 mg/L and atherosclerotic cardiovascular diseases, which can also help to reduce inflammation in VGD.16
A recent solution to preventing VGD is the use of extravascular support. The extravascular support acts as a protective outer layer of SVG, which minimises wall stress and reduces activation of SMC and VEC by a stretch mechanism. Upon external stenting, the VEST trial showed a decline in lumen irregularity 4.5 years after CABG, as it reduced the remodelling of the SVG and greatly decreased the diffuse intimal hyperplasia. A significant factor appears to be close approximation of the external stent to the wall of the SVG. Further large randomised controlled trials are needed to refine the principle of extravascular graft support to boost the long-term patency of SVG.17
In-Stent Restenosis and Saphenous Vein Graft-Venous Graft Degeneration Intervention
Mechanisms of in-stent restenosis
Restenosis is a pathophysiological form of reaction to injury which triggers the narrowing of the vessel because of negative vascular remodelling or neointimal hyperplasia. Similarly, balloon dilatation and deployment of the stent leads to damage to the vessel wall and triggers a healing response consisting of inflammation and proliferation of the VEC and SMC. A maladaptive healing response from the vessel wall leads to the development of various forms of ISR.18 Various stent designs and materials have proved to have a beneficial impact on the endothelial and medial layer damage caused by stenting. The higher the artery expansion achieved during balloon inflation, the greater the damage sustained on the media layer, leading to a greater ISR rate. Therefore, the production of ISR during stent deployment is directly related to the magnitude of artery expansion.19 He et al.19 created a tissue-growth model, which compares the stent-induced tissue damage with the ISR and using the finite-element method to simulate ISR creation after PCI.
Techniques to tackle in-stent restenosis
Various stent designs and materials have proved to have a beneficial impact on the endothelial and medial layer damage caused by stenting. With respect to the materials used to make stents, the ISR was lower for softer materials than for harder ones. However, for polymeric stents, the need of bulkier stent design to satisfy the strength requirements may cancel out such gains. A newer-generation, ultrathin (60 µm) DES-biodegradable polymer-coated SES has been designed to substantially minimise the ISR and MACE, including composite of cardiac death, myocardial infarction, and target-lesion revascularisation.20
Another possible cause for ISR is the premature, untimely release of cytostatic drugs, which contribute to overdose and further delay the restoration of the endothelium.21 The antiproliferative drugs have no specificity, which not only inhibits the proliferation of vascular SMC, but also blocks the proliferation of VEC and slows the endothelialisation in an inverse relationship.
Techniques for optimising drug dissolution
The pattern of drug release has a significant effect on its retention in the arterial wall, which can affect healing of the blood vessels and therapeutic effects. Various other novel strategies for regulating the rate of drug release leading to controlled delivery, as well as other, newer stent platforms, are summarised in Figure 3 and discussed below.
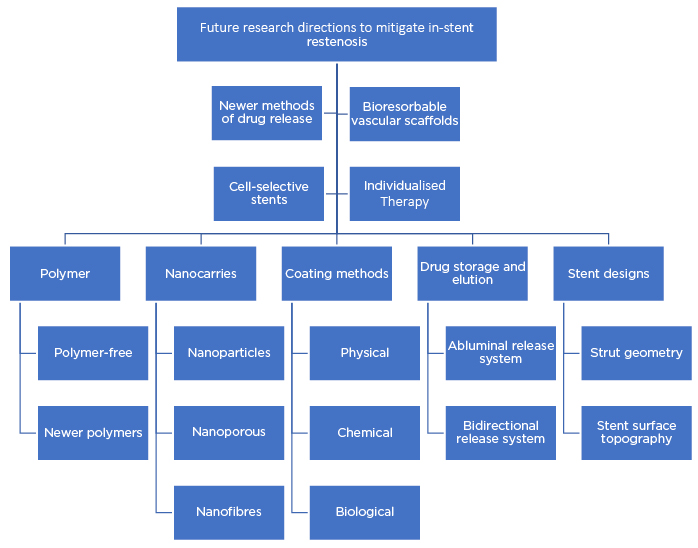
Figure 3: Flow chart summarising the future research strategies to mitigate in-stent restenosis.
Polymers
Permanent and biodegradable polymers are commonly used in present generation DES, which could result in ISR and late stent thrombosis. Polymer-free DES have better drug delivery methods and effectively prevent ISR.22 DES without a polymer can minimise the risk of polymer-induced late-stage thrombosis, which also has promising drug dissolution rate and effectively prevents ISR.
New polymers have effective drug dissolution mechanisms that match physiological properties such as temperature and bioactive surfaces, and mechanical properties such as alternating multiblock structure.23 Nanostructured hybrid polymers, called polyhedral oligosilsesquioxane thermoplastic polyurethanes, control the release of drug and can be effective. Nanocomposite polymers include polyhedral oligomeric silsesquioxane poly (carbonate-urea) urethane, which serves as a framework for the attachment of biologically active compounds, used for DES coating materials with covalently linked anti-cluster of differentiation (CD)34 antibodies.
Nanocarrier technology
Because of their special biochemical properties, nanocarriers can deliver drugs inside the cells of the vessel wall. These nanoscale carriers store drugs using the core-shell technique, which releases drug in two phases: earlier immediate-release and later slow-release, combining new biologically absorbable stent materials.22,24,25 A substantial number of studies have shown nanoparticles are able to carry different drugs or biomolecules to achieve the effects of a predictable controlled release of drugs. Additionally, nanoporous materials enable drug dissolution by altering nanopore or nanotube structures and regulating microporous openings. Currently, nanotubular titanium and nanoporous anodic alumina are used as nanopores because of their exceptional inert, nontoxic properties. Nanofibers are another material that has unique characteristics, including small pores, high porosity, and a large surface area because the polymer nanofibers are electrospun into ultrafine fibres. Their high volume-to-surface ratio can boost drug loading abilities, cell attachment, and mass transfer.
Coating technologies
Appropriate surface transformation of the stent should prevent immune processes, the development of thrombosis, and intimal hyperplasia, and accelerate endothelialisation to form a continuous endothelium coating on the stent surface within the first month. There are various physical, chemical, and biological methods developed for the surface modification, including the preparation of stent grooves; the use of nanoscale carriers, which store drugs using the core-shell technique and leads to earlier immediate-release and later slow-release; and the combination of new, biologically absorbable stent material.22
Much of the development of new polymers and the advancement of coating technology has tried to capture molecules to trap the stem cells as a stent coating.23 Physical methods include electrostatic dry powder deposition technology, used to embed sirolimus in polyethylene-co-vinyl acetate (PEVA)/polybutyl methacrylate (PBMA) polymer microparticles, with an average diameter of 3 μm. Once these microparticles have been applied, they begin to melt on the stent surfaces to produce a homogeneous continuous film that displays continuous release over 25 days.26
Specific chemical methods include acid/alkaline anodic oxidation, often used for biphasic release processes in which firmly linked compounds are delivered from DES at a sustained rate along with the drug, while the weakly bound molecules are liberated in smaller spurts. The coating of polydopamine (PDA) exhibits a significant specificity for different substrates leading to secondary reactions such as different cell–material interactions that enhance endothelial cell proliferation and reduce SMC proliferation. It offers DES with a tangible and enticing surface-modifying approach.22,27 Another novel technique for improving stent surface haemocompatibility is the phosphorylcholine (PC)-based stent coating. Lewis et al.28 demonstrated that the PC-coated device supports its nonthrombogenic purpose and exceptional tolerance of the arterial wall, as well as the ability to activate a variety of therapeutic agents.
Many of the biological methods used are based on the basic surface markers of stem cells, which are not completely known, and how the stem cells differentiate directly into specific cells and the transformation pathways remain unclear; this is one of the major hurdles in further developing this technology.29
For the effective release of antiproliferative drugs to reduce ISR, various directive delivery systems methods have been established.30,31 The abluminal release system involves the antiproliferative drug being only coated on the abluminal or external surface of the stent to allow the luminal or internal surface to be a bare-metal surface (also called a mural-release system). It aids reduction of polymer exposure to the luminal area of the blood vessel, which allows the drug–polymer matrix to interact only with the blood vessel wall. A bidirectional release system is a two-sided coating method in which the antithrombotic/endothelisation-promoting drug can be layered on the luminal surface and the antiproliferative drug can be instilled on the abluminal surface.
STENT DESIGN
Advances in strut structure and topography of the stent surface have resulted in improvements in the cross-sectional design, influencing the consistency of the flow pattern and thus reducing the deposition of prothrombotic fibrin and promoting the production of endothelial anticoagulant thrombomodulin, which prevents late stent thrombosis.32
Progress in micro- and nanotechnology has made it possible to produce fine configurations on metal and polymeric biomimetic coatings that are simple and cost-effective. Polymer-free DES uses techniques of multiple reservoirs, grooves, or micropores in the stent struts to load the drug, which is released directly to the arterial wall and would not get flushed away by the circulation.33
The various nanotopography geometries, such as nano-gratings, nano-post arrays, nano-islands, and nano-pits, modify the surface of the stent physically. These grooves and cavities are crafted on the stent struts by laser engraving. In this situation, the stent must have excellent biocompatibility and mechanical properties, with sufficient compressive strength and adaptability.22
Bioresorbable Scaffold
Long-term follow-up evidence from the leading bioresorbable scaffold (BRS) has raised concerns about its efficacy and safety because of the relatively high incidence of scaffold thrombosis. Clinical studies on bioresorbable metal scaffolds include the use of magnesium and its alloys.34 Magnesium metal is commonly used in coronary stents because of its excellent properties, including high resistance, outstanding reverberation and impact resistance, and good thermal and electrical conductivity.35 Research in this field is promising, as different improvised next-generation European Conformity (CE) mark-approved BRS have been designed and their implantation techniques further refined. The thinner strut is the latest generation of BRS and is the most innovative technology with the potential for overcoming the current limitations.36
Cell-selective antirestenosis-prohealing drug-eluting stents
As the mechanisms of action of the drugs eluted from the DES are nonselective, available therapies are not adequate for preventing ISR. To date, there are no cell-selective drugs
available which could differentiate between inflammatory and prohealing components. Canfield et al.37 demonstrated the effects of the experimental microRNA-based cell-selective strategy in their preclinical studies, showing inhibition of vascular SMC proliferation while promoting EC antithrombotic and anti-inflammatory functions.37,38 Such strategy forms the framework for developing innovative cell-selective DES, designed to eliminate the need for extended dual antiplatelet medication regimens.
Individualised Treatment for Coronary Artery Disease
The Human Genome Project (HGP) established the foundation for the development of precision medicine, also known as personalised medicine.37 Genome-wide association studies have
identified a wide range of single nucleotide polymorphisms that significantly raise an individual’s predisposition to coronary artery disease, ISR, and antiplatelet therapy response.39 Consequently, genetic screening can predict the PCI-related outcomes in patients, which could further individualise the therapy.40
CONCLUSIONS
Though SVG intervention itself is a complicated event, especially if it occurs many years after the CABG procedure, if the interventional procedure is performed with the use of appropriate hardware and with the necessary expertise, it can be easily intervened without any further complications or events. Accordingly, in this case, the patient was treated successfully with the use of the potent antiplatelet agent ticagrelor and the latest generation stent, without use of any additional protective devices. There are no case studies documenting the intervention of diffuse VGD in the literature. Newer therapies targeting the pathways of inflammation and cell cycle may improve the long-term patency of SVG and DES/scaffolds.
Declaration of Patient Consent
The authors certify that they have obtained appropriate patient consent. In the form the patient gave her consent for her images and other clinical information to be reported on and published. The patient understands that their names and initials will not be published and due efforts will be made to conceal their identity, but anonymity cannot be guaranteed.