Abstract
Stent thrombosis (ST) is uncommon yet constitutes the most feared complication following percutaneous coronary intervention. Although its incidence is now <1% within a year after stenting in patients receiving second or later-generation drug-eluting stents (DES), compared to those in the first-generation DES-era, the clinical impact of ST is still high, because the majority of cases with ST are complicated by critical consequences, including myocardial infarction and even sudden cardiac death. Moreover, the pathophysiology and risk factors leading to ST were recently re-recognised, as bioresorbable scaffolds (or biodegradable vascular scaffolds) have now been developed, and concerns have arisen regarding scaffold thrombosis, which serves as another ‘ST’. Accumulating evidence through the bare-metal stent and DES-era has identified clinical factors associated with increased risk of ST, such as patient-related, lesion-related, procedure-related, and post-procedure-related risk factors. Therefore, this short review describes updated pathophysiology and contributing risk factors for stent (or scaffold) thrombosis, which are useful for risk stratification in patients with coronary artery disease in the late metallic DES-era or at the beginning of the bioresorbable scaffolds era.
INTRODUCTION
The development and clinical introduction of metallic coronary stents in the late 1980s greatly reduced the risk of early adverse events, such as abrupt vessel closure, and in turn dramatically improved outcomes of patients who underwent percutaneous coronary intervention (PCI), compared to balloon angioplasty alone.1 Nevertheless, deployed intracoronary stents still led to substantial concerns regarding early and remote complications. Two major causes of coronary stent failure following PCI include in-stent restenosis (ISR) and stent thrombosis (ST). In the bare-metal stent (BMS) era, the angiographic restenosis rate was high, ≤15–30%,2 while the incidence of ST ≤1 year after stenting in patients with BMS and first-generation drug-eluting stents (DES) ranged from 0.6–3.5%.3,4 However, the recent incidence of both ST and ISR has been markedly reduced, mainly due to widespread use of post-second-generation DES combined with an optimised cocktail of antiplatelets for optimal duration. Recent large-scale registries and randomised trials demonstrate that ST occurs ˜1% through 1-year post-stenting and has a <0.5% annual incidence rate afterwards.5,6 With respect to mechanisms of early and late stent failure, high-resolution intravascular imaging, called optical coherence tomography (OCT), showed that neoatherosclerosis, defined as lipid-rich and calcified fibroatheroma, or fibrocalcific plaque within stented segments,7 is an important histopathological change for both ISR and ST.8 While ISR rarely leads to critically clinical consequences, such as cardiac death, ST is a serious, potentially life-threatening clinical event typically resulting in ST-elevation myocardial infarction, including a substantially high mortality rate ≤25% within 30 days.9,10 In recently developed strategies, other than implantation of metallic stents in the coronary artery for life, including drug-coated balloons or bioresorbable vascular scaffolds (BRS), PCI-related thrombosis is growing increasingly clinically important.11
In order to standardise the nomenclature on ST from a wide range of clinical trials, registries, and meta-analyses of coronary stents, a group of experts known as the Academic Research Consortium (ARC) proposed universal definitions in 2006 that are now widely accepted.12 This definition classified evidence of ST in accordance with certainty of clinical findings of ST as definite, probable, or possible, as well as timing after the indexed stent implantation, as early (≤30 days), late (31 days–1 year) or very late (later than a year), which may have distinct pathophysiologies. Moreover, early ST is further divided into two categories, including acute ST (≤24 hours) and subacute ST (>24 hours–30 days).
STENT THROMBOSIS IN THE BARE-METAL STENT ERA AND DEVELOPMENT OF DUAL-ANTIPLATELET THERAPY
The limitations of balloon angioplasty when it was introduced in 197713 included acute adverse events (induced by early abrupt vessel closure due to vascular injury without adequate acute gain), high rates of restenosis (due to the excessive healing process by activated smooth muscle cells), and accumulation of inflammatory cells against ballooninduced vascular injury with or without negative vascular remodelling.14 Since 1986, implantation of a metallic stent in the treated coronary arteries15 was a strategy for reducing acute complications of balloon angioplasty. However, at the beginning of the coronary stent era, a significant number of cases still continued to be complicated by ST. In short, after the introduction of stents to clinical practice, complete occlusion of stents occurred in >20% of cases, mostly within 14 days.16 However, procedures were performed with intensive use of anticoagulants, which resulted in critical haemorrhagic complications (major bleeding occurred in 9% of patients).17 Therefore, the most important and evolutional pharmacological development in PCI therapy was dual antiplatelet therapy (DAPT), the combination of aspirin and an adenosine diphosphate (ADP)-receptor (P2Y12) inhibitor, which dramatically reduced both early ST and bleeding complications. Accordingly, many randomised clinical trials demonstrated that DAPT was conclusively superior to anti-coagulation for the prevention of early complications after stent deployment.18 In combination with accumulating technical and procedural optimisations, such as high-pressure inflation following stent deployment and complete coverage of the plaque without edge dissection, these intensive antiplatelet therapies successfully facilitated the further widespread indication of PCI for the treatment of a broader range of coronary artery diseases when compared to balloon angioplasty.18
STENT THROMBOSIS IN THE DRUG-ELUTING STENT ERA
Despite the importance of radial force powered by metallic stents for avoiding early and late constrictive vascular mechanical forces, its deployment procedure using high pressure balloon induced acute vessel injury, which enhances the vascular healing process and in turn leads to significant neointimal hyperplasia via smooth muscle cell proliferation. ISR was the main issue that motivated research interest in the development of a novel type of metallic stent. DES, which slowly and locally release anti-proliferative agents including sirolimus, paclitaxel, biolimus, and everolimus, suppress in-stent neointima hyperplasia via the inhibition of smooth muscle activation and proliferation. They have been clinically proven to be dramatically effective in reducing the incidence of ISR, which presents as target lesion/vessel revascularisation in many clinical trials, and have enabled the expansion of PCI indications to treat the lesions of even high-risk patients, such as patients with relatively long and small diseased coronary vessels, multi-vessel disease, and lesions in the left-main coronary artery that used to be encouraged for coronary artery bypass grafting rather than PCI.
In spite of the obvious superiority of DES in preventing ISR and the need for repeat revascularisation,19 concerns regarding mainly late or very late ST have emerged due to the nature of DES, such as delayed endothelial cell proliferation and polymer-induced prolonged vessel wall inflammation followed by positive vascular remodelling, as well as late stent malaposition.20 To minimise these concerns, second-generation DES were developed by the use of technologies such as new biocompatible polymer coatings and thinner cobalt-chromium metal struts, and they showed significant superiority in comparison with first-generation DES, decreasing the risk of ST and ISR. The everolimus-eluting stent has been found to be safer and more effective than first-generation DES.21,22
PATHOPHYSIOLOGY AND MECHANISMS OF STENT THROMBOSIS
Recent large-scale registries show that, with contemporary antithrombotic therapies and modern-generation DES, the rate of early ST is relatively low, at <1%.23 Similarly, a systematic review of randomised trials with DES reporting results at 12 months after implantation showed a median incidence of definite ST of 0.61%.5,6 However, in spite of such a low incidence of ST in <1% of patients receiving stents, ST should not be underestimated, as critical consequences are highly likely once it occurs. Thrombus aspiration (thrombectomy) and balloon angioplasty are frequently performed in combination with repeat stenting in 30–50% of cases, as treatment of ST.24
It is widely known that ST occurs more frequently in complex patients and lesions, such as those with acute coronary syndromes, diabetes mellitus, chronic kidney disease and diffuse disease, small vessels, and bifurcation lesions requiring multiple stents. Even multifactorial, accumulating evidence indicates that mechanisms and pathophysiology leading to ST can be classified into four major categories: i) patient-related, ii) lesion-related, iii) procedure-related, and iv) medication (post-stenting)-related (Figure 1). As part of the patient-related factor, so-called complex patients are generally at high risk of ST, including those with smoking habits, diabetes, chronic kidney disease, and acute coronary syndromes with a large thrombotic burden. Additionally, this includes patients with thrombocytosis and with high platelet activity against antiplatelets.25 Understanding the risk of ST according to these patient-related factors facilitates the use of procedural strategies to minimise the risk of ST, and careful patient selection, lesion selection, device selection, and planning strategy of the procedure is the first critical step. Similar to patient-related risk factors, complex lesions are also at high risk for ST, including diffuse and long lesions, which require long stenting, small vessels, bifurcations, and lesions with thrombotic burden. Furthermore, recent significant OCT studies revealed various contributing lesion-related mechanisms of ST. While stent underexpansion and dissection at stent edges were associated with early ST,26 detailed analysis from an OCT registry revealed that findings of stent malapposition causing shear flow disturbance, neoatherosclerosis, and uncovered stent struts were risk factors for very late ST.27 Therefore, to reduce the risk of ST, final adequate lumen area in combination with good apposition of stent to arterial wall by high-pressure balloon is particularly essential. For that purpose, intravascular imaging using intravascular ultrasound or OCT is helpful, as they can provide significant insight regarding lesion preparation and selection of stents with appropriate sizing. Additionally, residual dissection in the stent edge is also a strong predictor for ST, especially when it limits coronary flow.
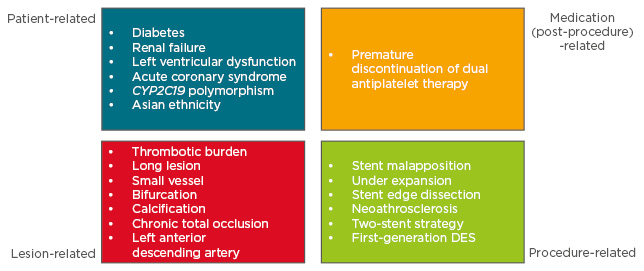
Figure 1: Risk factors associated with stent/scaffold thrombosis.
DES: drug-eluting stent.
EARLY STENT THROMBOSIS (≤30 DAYS)
Clinically and pathologically, ST can be classified into two major categories according to the timeline: early ST is within the first 30 days and late ST is beyond 30 days after index procedure. Early ST is further divided into acute (≤24 hours) and subacute (>24 hours–30 days). Early ST is more common than late ST, accounting for 70% of all ST cases.28 For early ST, lesion and procedure-related risk factors are relatively more important. Stent undersizing with incomplete stent apposition; presence of residual dissection; lesions with thrombotic burden, typically in settings of acute coronary syndromes; impaired coronary flow; and residual disease are all significant predictors of early ST.29,30 Other than these factors, premature discontinuation of DAPT in the initial 30 days after stenting is obviously the most critical predictor of early ST.31
LATE STENT THROMBOSIS (>30 DAYS)
Although procedural issues in stenting will more likely lead to early ST, these factors can also play essential roles in late ST, where mechanical issues of implanted stents, such as stent under-expansion with incomplete apposition and edge dissection, remain critical for ST even after the time point of DAPT discontinuation.29 Among these factors, incomplete stent apposition is frequently observed on intravascular imaging using OCT, rather than intravascular ultrasound, in patients with either early or late ST.32 Moreover, a number of metaanalyses showed evidence of a significant increase in the risk of ST with both first-generation sirolimus and paclitaxel-eluting stents, compared to newergeneration DES.3,33 In first-generation DES, delayed endothelial cell coverage of implanted stent struts induced a broad spectrum of pathological entities including not only ST but also delayed late luminal loss contributing to late restenosis, known as the ‘late catch-up phenomenon’,34 persistent vasomotor dysfunction,35 and de novo in-stent atherosclerosis.36 Induction of persistent inflammatory and thrombogenic reactions of stented vascular wall by biocompatible polymer coating of DES may cause adverse complications following DES deployment.37 Meanwhile, newer-generation DES seem to have substantially addressed this issue by incorporating thinner stent struts, biocompatible polymer coatings whether non-erodible or biodegradable, and lower dosages of sirolimus.5
CYP2C19 POLYMORPHISM AND STENT THROMBOSIS
Considerable interest has focussed on predicting ST risk based on response to ADP receptor (P2Y12) antagonists. Clopidogrel, a selective inhibitor of the platelet P2Y12 receptor, has been a standard antiplatelet treatment dominantly added to aspirin following PCI, and it is an inactive pro-drug that requires hepatic metabolism by cytochrome P450 2C19 (CYP2C19) into its active form. However, substantial subpopulations have been recognised to have an inadequate response to clopidogrel, which leads to insufficient antiplatelet effects and, ultimately, ST. Individuals carrying at least one loss-of-function allele (either *2 or *3) of the CYP2C19 gene demonstrate reduced active clopidogrel metabolites and suppressed antiplatelet activity.38 The frequency for the most common loss-of-function variant CYP2C19*2 is <15% in Caucasians and Africans, while affecting ≤35% of those of Asian descent. Accordingly, among East Asian populations in particular, the number of patients defined as a ‘poor clopidogrel metaboliser’ carrying the CYP2C19*2/*2 or *2/*3 polymorphisms may be higher than previously postulated. Platelet function monitoring can measure the platelet reactivity of individual patients and may be able to adjust antiplatelet therapy for better clinical outcomes. However, no randomised clinical trial has demonstrated benefits based on platelet reactivity.39-41 As an impaired response to P2Y12 antagonism also confers increased risk of ST,42 alternative P2Y12 inhibitors, such as prasugrel and ticagrelor, that are less influenced by polymorphisms of the CYP2C19 gene, may be encouraged for use primarily in patients planning PCI with high-risk lesions for critical consequences once ST occurs, such as patients requiring multi-vessel or left main coronary stenting, especially in East Asians (Figure 2).43 However, although the effects of P2Y12 inhibitors are potentially limited in East Asians, the total incidence of thrombotic complications after PCI is similar or even lower than Caucasians.44
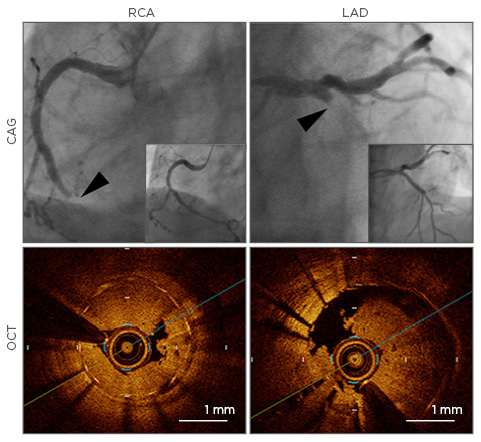
Figure 2: A case with simultaneous stent thrombosis in the right coronary artery and left anterior descending artery.
Upper: CAG at stent thrombosis (main figures) and after revascularisation with aspiration thrombectomy and balloon angioplasty (figures in right bottom corner); Lower: stent thrombosis in RCA and LAD revealed by OCT. Arrowheads indicate lesions of stent thrombosis.
RCA: right coronary artery; LAD: left anterior descending artery; CAG: coronary angiography; OCT: optical coherence tomography.
Adapted from Chikata et al.43
BIORESORBABLE SCAFFOLDS AND THROMBOSIS
As a new-generation device to treat coronary artery disease next to DES, BRS are an important technological innovation that will be a potential breakthrough for improving outcomes of patients with coronary artery disease, and may radically change future PCI strategies. Theoretically, once the implanted scaffold is fully degraded, the stented coronary segment/artery will be free of concerns regarding future stent failure, ST, and restenosis with complete restoration of normal vasomotor function. In clinical trials to assess efficacy of BRS, which compared everolimus-eluting BRS (Absorb, Abbott Laboratories, Chicago, Illinois, USA) to a metallic everolimus-eluting cobalt chrome stent (Xience, Abbott Laboratories) that is widely used as second-generation DES, the overall performance of BRS did not seem unsatisfactory for relatively short-term follow-up, consisting of 1 year in Europe and Asia (ABSORB III and ABSORB Japan trials).45,46 However, these trials did not seem to have enough power to detect the risk of scaffold thrombosis (scT) in patients receiving BRS. Moreover, 3-year outcomes from the ABSORB II trial, a prospective randomised trial that included 501 patients with one or two de novo coronary lesions, showed that treatment with BRS was associated with a two-fold increased risk of device-oriented clinical events, specifically an increased risk of target-vessel myocardial infarction, as well as an increased risk of late scT. There were six incidents of definite scT occurring beyond a year among patients who received the BRS, compared with no reported cases of definite or probable ST for patients who received the metallic DES. Additionally, vasomotor functions at 3 years were similar in both groups.45 A meta-analysis of six trials addressing the efficacy and safety of Absorb versus Xience showed a significantly higher incidence of ST, especially within the initial 30 days after deployment.47 Two-year results from the Amsterdam Investigator-Initiated Absorb Strategy All-Comers (AIDA) trial showed that use of BRS was associated with increased risk of scT, and of target-vessel myocardial infarction, compared to those receiving DES.48 The US Food and Drug Administration (FDA) recently announced increased incidence of major adverse cardiac, as well as thrombotic events in patients receiving BRS, compared to those with metallic DES based on results from the 2-year follow-up of the ABSORB III trial. The FDA recommends appropriate selection of target vessel/lesion and optimal device. Accordingly, optimal BRS implantation strategy has recently been referred to as ‘PSP’, referring to: Prepare the lesion, appropriate Sizing, and Post-dilatation. These findings suggest that ST after BRS implantation is more likely related to procedures, which the expertise of operators may largely affect, as implantation procedures are not very different to those in current metallic DES. Therefore, in the case of using the current version of BRS, careful patient selection and lesions in combination with efficient lesion preparation by balloon pre-dilatation, and appropriate scaffold deployment followed by sufficient post-dilatation aiming at good scaffold apposition, are critical. Moreover, for these purposes, detailed intravascular imaging, especially OCT, for optimisation of scaffold deployment, is strongly encouraged for avoiding scT. Thus, in addition to accumulation of procedural and technical tips for the delivery, further technological evolution developing thinner strut of the scaffold, strengthening radial force, and further effective delivery of antiproliferative drugs will likely be required before widespread clinical indication of BRS for more complex patients with complex lesions.
CONCLUSIONS
ST has aroused significant clinical attention along with the change of coronary metallic stents over time. Despite recent relatively low incidence, the clinical importance of ST has been recently reaffirmed, mainly due to a new era of treating coronary artery disease with BRS. For avoiding this most critical complication of PCI, establishment of careful and optimal revascularisation strategies in accordance with the complexity of patients and lesions, adequate lesion preparation and post-dilatation for good stent apposition without edge dissection, and, most importantly, selection of appropriate antiplatelet agents for appropriate duration even in consideration of genetic background is essential.