Abstract
Acute kidney injury (AKI) is a common complication of critical illness and is associated with high morbidity and mortality. The epidemiology and pathogenesis of AKI and changes in renal function and preventive strategies are areas of interest. Although the aetiology of AKI is often multifactorial, sepsis has been consistently found to be a leading contributing factor in AKI during critical illness. Despite revised guidelines and better haemodynamic management, the outcome of AKI is still a reason for concern. Critically ill patients with AKI have significantly improved short-time prognosis with current treatment standards but are more prone to develop increased morbidity in the near future.
INTRODUCTION
Acute kidney injury (AKI) is a common complication of critical illness and is associated with high morbidity and mortality.1 The epidemiology and pathogenesis of AKI, assessment of baseline and changes in renal function, and preventive strategies are particular areas of research interest. AKI is a syndrome that is characterised by a rapid decline in renal function and urine output, resulting in retention of waste products such as urea, nitrogen, and serum creatinine. Life-threatening consequences include volume overload, hyperkalaemia, and metabolic acidosis.2-4
The incidence of AKI in intensive care unit (ICU) patients is rising due to the population age increasing, more comorbidities, and a higher prevalence of risk factors. Improved ICU management has, however, significantly diminished morbidity and the mortality of patients who develop AKI. Despite an increase in the number and severity of comorbidities, in-hospital mortality has declined, but the incidence of AKI and AKI requiring renal replacement therapy (RRT) has increased over time.5 In a retrospective cohort study, Hsu et al.6 recently identified diagnoses or procedures that may be a driver for the risk in dialysis-requiring AKI. Results showed that the temporal trend in acute or chronic diagnoses: septicaemia, hypertension, respiratory failure, coagulation/ haemorrhagic disorders, shock, and liver disease accounted for the progressive trend in dialysis- requiring AKI. In contrast, temporal trends in surgeries (e.g. cardiovascular surgery, non-kidney solid organ transplantation) or procedures (e.g. respiratory intubation/mechanical ventilation) commonly associated with dialysis-requiring AKI did not account for the increasing dialysis-requiring AKI trend.6
Although these results are interesting, they should be interpreted with caution. Some diagnoses or procedures can be influenced by multiple factors (such as comorbidity, difference in critical care, prevention of nephrotoxic injury, and use of AKI definitions), which can differ between hospitals. Heart failure, age-related structural changes, and functional changes in the kidney can increase the risk for AKI; however, the extent to which these increases reflect changes in underlying patient characteristics, provider practices, or increased availability of RRT over time is not yet known.7
Utilisation of RRT to support ICU patients with AKI is growing.8 A more liberal application of dialytic support could explain the increasing incidence of AKI requiring RRT. Other possibilities include the increasing availability of RRT and earlier or lower thresholds for initiation; however, to date there is no consensus about an early start or ‘wait and see’ approach.7 Furthermore, recent practice surveys suggest that nephrologists are more likely to initiate RRT based on more imminent indications, such as hypervolaemia, acidosis, or electrolyte disturbances, rather than the degree of azotaemia alone, particularly as the severity of illness increases.7,9
EARLY RECOGNITION OF ACUTE KIDNEY INJURY IN THE CRITICALLY ILL
In 2004, the Acute Dialysis Quality Initiative (ADQI) group, which represents nephrology and critical care specialists, proposed the Risk, Injury, Failure, Loss of kidney function and End-stage renal disease (RIFLE) criteria to define AKI.2 RIFLE includes two separate criteria for renal failure: changes in serum creatinine (SCreat), changes in urine output (UO), or both. RIFLE defines three levels of increasing severity of AKI (i.e. Risk, Injury, and Failure) and two outcome classes (i.e. Loss and End-stage renal disease).
In 2007, the Acute Kidney Injury Network (AKIN) refined this approach and proposed some small modifications to the RIFLE criteria. Briefly, relatively minor changes in SCreat occurring within a 48-hour window were associated with significant risk of adverse outcome. According to the new definition, the RIFLE-R category was broadened (increase in SCreat of ≥0.3 mg/dL even if a 50% threshold was not attained) and patients were categorised as ‘failure’ when they received RRT, regardless of SCreat values or UO at initiation. The AKIN also proposed the use of Stages 1, 2, and 3 instead of the categories R, I, and F.10-12
The Kidney Disease: Improving Global Outcomes (KDIGO) working group recently combined the RIFLE and AKIN classifications to establish one internationally accepted AKI classification for clinical, research, and public health use. KDIGO takes changes in creatinine within 48 hours, or a decline in the glomerular filtration rate (GFR) over 7 days, into account. AKI is defined as an increase in sCreat ≥0.3 mg/dL within 48 hours, or an increase in sCreat ≥1.5-times baseline, which is known or presumed to have occurred within the prior 7 days, or a UO of <0.5 mL/kg/h for 6 hours.13
However, despite improvements of definition, renal function should be measured and monitored in real time so that a decline in renal function and the occurrence of AKI is visible as soon as possible. However, the diagnosis of AKI is based on SCreat rise and/or fall in UO, two markers which are not renal specific and have important limitations.14
The reported incidence of AKI in the literature varies substantially with the population evaluated and the definition used and the importance of the oligo-anuria component is thoroughly covered. Koeze et al.15 assessed which of the AKI definitions, with or without UO criteria, recognised AKI most rapidly and frequently. They concluded that AKIN and KDIGO criteria detect more patients with AKI compared to RIFLE criteria. The addition of UO criteria helps to detect patients with AKI 11 hours earlier than SCreat criteria and may double AKI incidences in the critically ill.15 It was concluded by Leedahl et al.16 that 3–5 hours of consecutive oliguria in patients with septic shock may provide a valuable measure of AKI risk. Although this trial is a retrospective analysis, it did show that duration of oliguria is of high importance in the development of septic AKI.16
TYPES OF ACUTE KIDNEY INJURY
There is still some dispute over the characterisation of the different types of AKI. Classically there are three types of AKI: pre-renal, intrinsic renal, and post-renal failure. These are characterised as decreased renal blood flow (in 40–70% of the patients), direct (intrinsic) renal parenchymal damage (in 10–50% of the patients), and obstruction of urine flow, which is less common in the ICU (10%), respectively.3,17
According to this classification, pre-renal AKI represents a separate entity characterised by a rapidly reversible increase in SCreat and urea concentration altering glomerular filtration, without primary parenchymal disease. This can be seen as adapted renal responses to a variety of negative stimuli. Pre-renal AKI and acute tubular necrosis (ATN) can exist simultaneously in the same patient. It is possible that some regions of the kidney can have severe morphologic and functional ATN, whereas other parts may be structurally intact, requiring only reperfusion to resume normal filtration. Therefore, AKI should be seen as a continuum between pre-renal, without structural injury, AKI, and AKI with renal injury, such as ATN, and in this instance the term transient AKI can be used. The aetiology and prognosis of transient AKI is arbitrarily defined as AKI of ≤3 days duration. A kidney biopsy can be helpful in excluding rapidly progressive glomerulonephritis, vasculitis, and interstitial nephritis.18
In the absence of pathophysiological findings of a renal biopsy, pre-renal disease can be distinguished from ischaemic or nephrotoxic ATN by examination of the urine. The kidney varies the rate of sodium excretion to maintain effective circulating volume. This response is mediated by a variety of factors including the renin-angiotensin-aldosterone system and possibly atrial natriuretic peptide. The urine sodium concentration can be used as a measure of volume status. A urine sodium concentration below 20 meq/L is indicative of hypovolaemia or a pre-renal origin of AKI. In ATN, the urine sodium concentration usually exceeds 40 meq/L because of tubular damage, not reaching maximum sodium reabsorption. Determination of fractional excretion of sodium (FeNa) and urine osmolality also help to differentiate between pre-renal and intrinsic renal AKI.
A low urine sodium concentration points to hypovolaemia, whereas a high value suggests ATN. However, a urinary sodium concentration of 20–40 meq/L can be seen with either disorder. This overlap can be differentiated by calculating FeNa. Sodium reabsorption is enhanced in hypvolaemic states leading to a FeNa <1% (99% of the filtered sodium has been reabsorbed), whereas tubular damage produces a FeNa >2%.19
The use of FeNa as a marker of tubular injury may be questioned. At the onset of sepsis and endotoxaemia, arterial vasodilatation is associated with stimulation of the renin-angiotensin system, arginine vasopressin release, and activation of the sympathetic nervous system. The resulting renal vasoconstriction is associated with an early increase in tubular sodium reabsorption leading to a decrease in urinary sodium concentration and FeNa.
FeNa values vary with respect to timing of measurement from onset of sepsis-induced tubular necrosis. This may explain why FeNa measurements range from very low to high in the more ‘controlled’ animal setting.20 Moreover, prolonged renal vasoconstriction during endotoxaemia will cause tubular dysfunction that is associated with increased cytokine, chemokine, and oxidant induced injury. This tubular damage converts a decreased FeNa into an increased FeNa which is dependent on the severity of sepsis and endotoxaemia. Thus, depending on the negative stimuli, FeNa may increase from <1% to >1%.21
Although urinary biochemistry could be an opportunity to explore the underlying diagnosis, the use of urinary examination is controversial and has not yet been validated. Many factors may lead to variable FeNa values during the day, which calls into question the usefulness of urinary biochemistry in daily practice. As a result, most studies were not able to find a consistent role for measurement of FeNa and/or fractional excretion of urea (FeUr).22 Vanmassenhove et al.23 have shown that in septic patients a low FeNa and FeUr is highly prevalent in the first hours of sepsis. A combination of a high FeNa and a low FeUr is associated with intrinsic AKI, whereas a combined high FeNa and FeUr is strongly predictive of transient AKI.23
BIOMARKERS
Currently, the standard diagnostic tools for AKI detection are monitoring of SCreat and UO, both of which are markers of renal function but not of kidney injury. SCreat is a delayed and insensitive biomarker of changes in kidney function and does not differentiate structural kidney damage and functional haemodynamic triggers and can be altered by a variety of factors. In addition, patients with reduced muscle mass may not have a robust rise in SCreat despite a substantial kidney injury.24 Biomarkers of AKI should have the ability to allow early detection of patients who are going to develop AKI. Furthermore, biomarkers should assist in the evaluation of the intensity of injury, differential diagnosis, and the impact of interventions on the recovery from kidney injury.
Within the past two decades, potential novel biomarkers measurable in urine or plasma of patients with AKI have been identified, including neutrophil gelatinase associated lipocalin (NGAL), kidney injury molecule 1 (KIM-1), interleukin 18 (IL-18), liver-type fatty acid-binding protein (L-FABP), tissue inhibitor of metalloproteinase 2 (TIMP-2), insulin-like growth factor binding protein 7 (IGFBP7), calprotectin, urine angiotensinogen (AGT), and urine microRNAs. However, the aforementioned biomarkers are not specific for AKI.24
Using a combination of two new markers, TIMP-2 and IGFBP7 (NephroCheck, Astute Medical Inc., San Diego, California, USA), appears to improve the identification of patients at risk of AKI at 12 hours compared with previous biomarkers.24,25 TIMP2*IGFBP7 measured early in the setting of critical illness may identify patients with AKI at increased risk for mortality or receipt of RRT over the subsequent 9 months.26 However, the challenge for the usefulness of biomarkers remains. This is not only in the early detection of AKI but also in the question of whether biomarkers can improve the outcome of AKI.
The same questions arise for the use of automated electronic alerts (e-alerts). E-alerts configured from electronic medical records and clinical information systems to warn healthcare providers of early or impending AKI have been evaluated.11 Recently, Lachance et al.27 reviewed all literature regarding e-alerts for AKI and concluded that e-alerts are heterogeneous in design, variably implemented, and seldom include clear direction for decision support. E-alerts for AKI appear not to improve patient outcomes, lead to improved utilisation of health services, or reduce RRT utilisation.27
RISK FACTORS
Although global care of critically ill patients has improved, AKI still carries a mortality rate of 50–90%.28-30 Depending on different clinical settings, such as post-cardiac surgery, contrast media exposure, severe heart failure with low output, and sepsis, pathophysiology and clinical features of AKI will differ and aetiology is multifactorial in most cases. It has been suggested that CKD is a risk factor for AKI because chronically impaired kidneys lose their ability to auto regulate, and therefore become susceptible to AKI whenever exposed to a sufficiently severe stimulus.31-33 Bedford et al.34 suggest that both AKI and CKD are not separate disease entities but are in fact components of a far more closely interconnected disease continuum. Considerable conceptual overlap may exist between these two separate conditions with regard to underlying pathology and pathophysiology, definitions, risk factors, and clinical outcomes. However, the true nature of this relationship is complex and poorly understood.34 The pathophysiology of AKI represents a very complex interplay between the immune system, the accompanying inflammatory response, tubular injury and the extent of any associated vascular insult.31,35
Ischaemia, inflammation, and direct toxic injury to the kidney are all major areas that contribute to the pathogenesis of AKI with significant overlap. Furthermore, epidemiological studies in AKI patients have determined additional risk factors such as age, hypertension, diabetes mellitus, and heart failure.32,36 Similar risk factors have also been identified for CKD. Prospective trials, such as the US-based ASSESS-AKI study, and the UK-based ARID study, are likely to shed new light on the relationships between AKI and CKD in the near future.31
Critically ill patients receive a myriad of medications. In the ICU, nephrotoxic drugs and antibiotics that reach toxic levels are responsible for 19–25% of AKI cases.37 Comorbidities known to significantly enhance the risk for drug-induced nephrotoxicity are underlying AKI or CKD, sepsis, advanced cirrhosis, liver failure, acute or chronic left heart failure, and various malignancies. Drug-related renal injury may be caused by haemodynamic instability, altered drug pharmacokinetics, direct renal parenchymal injury, or a combination of these factors.37 All phases of drug pharmacokinetics are disturbed in critically ill patients, including absorption, distribution, metabolism, and clearance. These changes often result from organ dysfunction, the acute-phase response of the underlying critical illness, multiple drug interactions, intravenous fluids, diagnostic procedures, and various medications.37
Although the aetiology of AKI in critically ill patients is often multifactorial, sepsis has been consistently found to be a leading contributing factor in AKI during critical illness.4,38,39 Because of the complexity of sepsis and AKI, it should be noted that no single pathway can explain all the features of septic AKI. Each septic AKI patient moves along an individual disease trajectory; therefore, the therapeutic targets vary with the underlying pre-existing conditions, time course, disease trajectory of sepsis, and AKI.33
In contrast to AKI, sepsis has benefited from a consensus-driven standardised definition for over a decade. Recently, the definition of sepsis was updated and validated (Table 1).40 Multicentre European studies found that AKI was attributable to sepsis and/or septic shock in 41.4–45.5% of critically ill patients.41,42 Parmar et al.43 proposed to define septic AKI as the simultaneous presence of both sepsis and AKI, in the absence of other clear and established, non-sepsis-related precipitants of AKI, for example, urinary tract obstruction, radiocontrast dye, and other nephro-toxins.43 The discrimination between septic and non-septic AKI may have clinical relevance. Septic AKI is characterised by distinct pathophysiology and has different clinical outcomes and responses to interventions compared with non-septic AKI. AKI has a negative impact on the long-term mortality of hospital-surviving septic patients.4,44,45
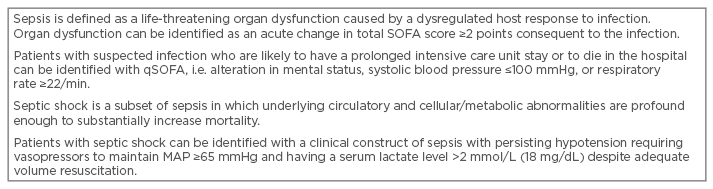
Table 1: Definition of sepsis and septic shock.40
MAP: mean arterial pressure; qSOFA: quick Sepsis Related Organ Failure Assessment.
Adapted from Singer et al.40
Observational data highlighted that septic AKI occurs more commonly among elderly and female patients compared to non-septic AKI. Septic AKI patients are also more likely to have a higher burden of pre-existing comorbidity compared with patients with non-septic AKI. In particular, septic AKI patients have a higher prevalence of congestive heart failure, chronic obstructive pulmonary disease, CKD, liver disease, diabetes mellitus, active malignancy, and immune system disorders and are more likely to be admitted for medical indications.43
The duration of hypotension before initiation of effective microbial therapy is a critical determinant of survival in human septic shock.46 Despite this clear observation, the literature on the exact haemodynamic goals to be applied in a clinical setting is scarce. There are no randomised control studies on the effects of different blood pressure levels on outcome to date. However, limited data from small cohort studies suggest a slight consensus on the use of arterial blood pressure targets in sepsis, and the preferred target range of 65–75 mmHg.47 Dünser et al.48 investigated the association between the arterial blood pressure during the first 24 hours and mortality in sepsis and concluded that a mean arterial pressure (MAP) level ≥60 mmHg may be as safe as higher MAP levels during the first 24 hours in septic patients. The group also hypothesised that although a MAP of 60 mmHg does not influence mortality, a higher MAP may be required to maintain kidney function.48 This hypothesis is confirmed by our study, which showed a significant influence of severe hypotension (<65 mmHg) on the evolution to failure.49
The FINNAKI study reported on the haemodynamic variables and progression of AKI in critically ill patients with severe sepsis.50 This prospective, observational study investigated the progression of AKI within the first 5 days of ICU admission, defined as new onset or worsening of AKI, according to the KDIGO guidelines, for both the AKIN/RIFLE classifications. They concluded that patients with progression of AKI had significantly lower time-adjusted MAP, 74.4 mmHg (68.3–80.8), than those without progression, 78.6 mmHg (72.9–85.4), p<0.001. A cut-off value of 73 mmHg for time-adjusted MAP best predicted the progression of AKI. Moreover, only the duration of a MAP <60 mmHg had a highly significant poor effect on the progression of AKI.50
Patients with an increased risk of AKI require careful attention for their haemodynamic status. First, hypotension results in decreased renal perfusion and, if severe or sustained, may result in kidney injury. Second, the injured kidney loses autoregulation of blood flow, a mechanism that maintains relatively constant flow despite changes in pressure above a certain point (mean: ~65 mmHg).51 Management of blood pressure and cardiac output require careful titration of fluids and vasoactive medication. Vasopressors can further reduce blood flow to the tissues if there is insufficient circulation of blood volume. However, early fluid resuscitation in the management of hypotensive patients with septic shock has been a standard treatment paradigm for decades. It has not been made clear how much fluid to give, for how long, or what type of fluid therapy is optimal in the physiologic support of septic shock, except avoiding synthetic starches. It is recommended by the updated international guidelines for management of septic shock that, in the resuscitation from sepsis-induced hypo-perfusion, at least 30 mL/kg of intravenous crystalloid fluid be given within the first 3 hours (strong recommendation, low quality of evidence). Following initial fluid resuscitation, additional fluids should be guided by frequent reassessment of haemodynamic status.52,53
Conversely, patients with AKI are also at increased risk of fluid overload and continued fluid resuscitation, despite increased intravascular volume, can cause harm.51,52 In resuscitated, critically ill patients, the distribution volume of SCreat is higher, which may lead to underestimating the severity of AKI. Fluid overload can be managed by diuretics, but the role of diuretics during septic shock is still controversial.54 Therefore, the working group for critical care nephrology made some recommendations for clinical practice: i) controlled fluid resuscitation in true or suspected volume depletion, ii) MAP >60–65 mmHg, yet target pressure should be individualised whenever possible and particularly when pre-morbid blood pressure values are known, iii) correction of vasoplegic hypotension in sepsis requires the use of norepinephrine as first-line therapy with vasopressin as a second-line agent along with fluid resuscitation.53
Next to critical care nephrology and the management of sepsis, it is necessary to gain further advances in targeted therapies in sepsisassociated AKI (SA-AKI) to improve outcome. Today, there is no effective therapy that has been shown to alter the outcome of SA-AKI.52 Human recombinant alkaline phosphatase (RecAP) is one of the limited pharmaceutical treatment options for SA-AKI currently being tested in a clinical trial setting. AP is a dephosphorylating, membrane-bound, endogenously occurring enzyme, exerting detoxifying effects through dephosphorylation of endotoxins, involved in sepsis pathogenesis. Although the mechanism of action is not completely understood, previous clinical trials in healthy volunteers and patients with sepsis, with or without AKI, have established the tolerability and potential efficacy of purified bovine intestinal AP (biAP). In patients with SA-AKI, biAP significantly improved renal function according to the combined endpoint of endogenous creatinine clearance, requirement for RRT, and duration of RRT.
Moreover, a range of markers of systemic inflammation, renal function, and renal damage in blood and urine demonstrated improvement, suggesting that a systemic anti-inflammatory effect induced by biAP prevented further renal injury. Following these encouraging results, a human recombinant AP (recAP) has been developed as a pharmaceutically acceptable replacement for bovine-derived AP. In line with preclinical and clinical studies using purified biAP, animal studies with recAP revealed potent anti-inflammatory activity preserving function and histological integrity of the affected kidneys (unpublished data) and no safety concerns were raised when administered to healthy volunteers. Therefore, a randomised, double-blind, placebo-controlled, four-arm, proof-of-concept, dose finding adaptive Phase IIa/IIb study was conducted and is still recruiting critically ill patients with SA-AKI.55
RECOVERY OF KIDNEY FUNCTION AND BEYOND
Recovery of kidney function is increasingly recognised as an important determinant of morbidity. Oppert et al.42 found that patients with pre-existing non-dialysis dependent CKD had a lower mortality as compared with septic AKI patients without pre-existing CKD.
Evaluation of a database of >40,000 critically ill patients highlighted that patients with presumed sepsis, advanced age, and underlying renal dysfunction had an increased risk for AKI regardless of having another organ failure at the time of ICU admission. Although proper management of AKI will improve outcomes for both low-risk (i.e. no respiratory failure or circulatory shock) and high-risk patients, there may be additional benefit for low-risk patients because their short-term outcomes (30-day ICU and hospital mortality) are more significantly impacted by AKI. This also emphasises the need for care after ICU discharge in an attempt to improve the patients’ outcomes and for assessment of potential recovery of renal function. Unfortunately, clinical follow-up of AKI survivors is low.56
Coca et al.57 carried out a meta-analysis of long-term renal and non-renal outcomes in patients with AKI. The pooled incidence of CKD and end-stage renal disease (ESRD) were 25.8 per 100 person-years and 8.6 per 100 person-years, respectively. Patients with AKI had higher risks for developing CKD (pooled adjusted hazard ratio [HR]: 8.8; 95% confidence interval [CI]: 3.1–25.5), ESRD (pooled adjusted HR: 3.1; 95% CI: 1.9–5.0), and mortality (pooled adjusted HR: 2.0; 95% CI: 1.3–3.1) compared with patients without AKI. The relationship between AKI and CKD or ESRD was graded based on the severity of AKI, and the effect size was dampened by decreased baseline GFR. This review demonstrates an association between AKI and CKD, because AKI was identified as an independent risk factor for CKD, ESRD, death, and other important non-renal outcomes (e.g. risk for cardiovascular disease and congestive heart failure).57 The long-term risk for cardiovascular events due to AKI was recently confirmed by a meta-analysis by Odutayo et al.58 AKI was associated with an 86% increased risk of cardiovascular mortality and with a 15% increased risk of stroke.
Because of an increased cardiovascular risk, life expectancy in survivors of AKI and critical illness is compromised. However, according to a small follow-up trial of those who survived AKI and recovered from RRT it seems that these patients have a satisfactory quality of life. Regular follow-up examinations should therefore be recommended by the attending physicians at hospital discharge.59
Regarding RRT, two main issues remain: the timing and the dialysis modality. The optimal timing of RRT in critically ill patients with AKI remains uncertain.60 Recently, two trials regarding initiating RRT with conflicting results have been published.61,62
In the ELAIN trial, Zarbock et al.61 investigated whether early initiation of RRT in patients who are critically ill with AKI reduces 90-day all-cause mortality. In this randomised clinical trial, 231 critically ill patients with AKI KDIGO Stage 2 (≥2 times baseline or UO <0.5 mL/kg/h for ≥12 hours) and plasma neutrophil gelatinase-associated lipocalin level >150 ng/mL were enrolled. Patients were divided into three groups: early start of RRT (within 8 hours of diagnosis of KDIGO Stage 2), delayed start of RRT (within 12 hours of Stage 3 AKI), or no initiation of RRT.61
Ninety-day mortality was 39.3% for patients undergoing early initiation of RRT compared to 54.7% for patients with delayed initiation (HR: 0.66; 5% CI: 0.45-0.97); more patients (53.6%) in the early group recovered renal function by Day 90 versus 38.7% in the delayed group.61
Gaudry et al.62 concluded in their randomised trial with AKI patients KDIGO Stage 3 divided into two groups: early strategy when RRT therapy was started immediately after randomisation or patients following the delayed strategy, when RRT was initiated if at least one of the following criteria was met: severe hyperkalaemia, metabolic acidosis, pulmonary oedema, blood urea nitrogen level >112 mg/dL, or oliguria for >72 hours after randomisation, that the mortality at Day 60 did not differ significantly between the early and delayed strategies, early-strategy group (48.5%; 95% CI: 42.6–53.8), and delayed-strategy group (49.7%; 95% CI: 43.8–55.0; p=0.79). The rate of catheter-related bloodstream infections was higher in the early-strategy group than in the delayed-strategy group (10% versus 5%; p=0.03). Moreover, diuresis (a marker of improved kidney function) occurred earlier in the delayed-strategy group (p<0.001).
Early RRT facilitates better fluid balance and electrolyte and acid base homeostasis and may remove circulating toxins and inflammatory cytokines during sepsis. Initiating RRT is not free of risks, which was agreed in the study of Gaudry et al.62 Both trials included patients according to the KDIGO criteria for AKI; however, it should be noted that patients in Stage 1, 2, or 3 normally do not receive RRT. Initiating RRT was part of the trial, but in daily life starting RRT is a decision, which is based on patient’s individual clinical status and dependent on more factors than only the KDIGO criteria; however, the trial of Gaudry et al.62 may support the wait and see approach.
Finally, if the decision is made to start with RRT, different modalities are available to provide RRT in ICU, including intermittent RRT (haemodialysis), hybrid therapies (i.e. sustained low efficiency dialysis, extended daily dialysis, prolonged intermittent RRT, continuous RRT [CRRT], and peritoneal dialysis). CRRT and intermittent RRT are generally considered complementary therapies with no clear evidence that either modality has a survival advantage. Selecting the optimal RRT modality should consider both patient specific characteristics (i.e. multi-morbidity, acuity, multi-organ failure) and ICU-specific operational characteristics (e.g. expertise to deliver prescribed therapy, resources). Intermittent RRT may be preferable when mobilisation and rehabilitation are a priority, provided metabolic fluctuations and fluid shifts can be tolerated.63 CRRT may be the preferable initial therapy over intermittent RRT particularly in clinical circumstances, for example, haemodynamically instable patients and acute brain injury. However, whether CRRT or intermittent RRT improves outcome and renal recovery remains uncertain.63 Anticoagulation strategy, choice of filter, and type of catheter are mainly subject of clinical judgement.
Next to the controversy in initiating RRT, the decision whether, or when, to stop RRT in a patient with AKI is still a subject of discussion.64 Withdrawal or withholding of RRT needs reflection regarding improvement of sufficient kidney function in relation to demand, improvement of the disorder(s) that prompted kidney support, and futility.
CONCLUSIONS
The kidney is an organ that can tolerate exposure to several negative stimuli without suffering significant structural or functional change. For this reason, any acute change in kidney function often indicates severe systemic derangement and predicts a poor prognosis. Risk for AKI is increased by exposure to factors that cause AKI or the presence of factors that increase susceptibility to AKI. Despite revised guidelines and better haemodynamic management, the outcome of AKI is still a reason for concern. Critically ill patients with AKI have better short-time prognosis than before but are more prone to develop increased morbidity in the nearby future. Therefore, any strategy in the critically ill with AKI requires consideration of each individual patient’s prospects on cure, care, and comfort.