Abstract
Metabolic acidosis is characterised by a primary decrease in the serum bicarbonate concentration, a secondary decrease in the arterial partial pressure of CO2, and a reduction in blood pH. Metabolic acidosis, acute or chronic, may have deleterious effects on cellular function and cause increased morbidity and mortality. A systematic review of the available literature was performed to identify data on the prevalence, manifestations, cause, outcomes, and treatment of metabolic acidosis in children. Online databases (Ovid Medline, Embase, and PubMed), commercial search engines (including Google), and chapters on metabolic acidosis in the standard textbooks of paediatrics and medicine were reviewed.
Systematic approach to acute metabolic acidosis starts with proper history taking and examination. This is followed by assessment of acid-base parameters, including pH, partial pressure of CO2, and bicarbonate concentration in arterial blood. Blood gas is needed to differentiate primary metabolic acidosis from compensated respiratory alkalosis. Once the diagnosis of a metabolic acidosis has been confirmed, serum electrolyte values are used to determine the serum anion gap. The various causes of increased and normal anion gap metabolic acidosis have been discussed in the article. The main aim of treatment in metabolic acidosis is to reverse the primary pathophysiology. In acute metabolic acidosis, sodium bicarbonate therapy is not beneficial due to potential complications and is reserved for specific situations. Base therapy is used in chronic metabolic acidosis where it ameliorates many of its untoward effects. Other modalities of treatment of metabolic acidosis include peritoneal or haemodialysis and tris-hydroxymethyl aminomethane.
Key Points
1. Metabolic acidosis is frequently observed in clinical practice, especially in patients who are critically ill and/or have renal failure.
2. Complex mechanisms are involved; in most cases, they are identifiable by medical history, pathophysiology-based diagnostic reasoning, and measures of some key acid-base parameters, which are easily available or calculable.
3. The therapeutic approach should be first aimed at early correction of concurrent clinical problems (e.g., fluids and haemodynamic optimisation in case of shock), in parallel to the formulation of a diagnosis. In case of severe acidosis, the administration of alkalising agents should be carefully evaluated, taking into account the risk of side effects, as well as the potential need of renal replacement therapy.
INTRODUCTION
Acid-base disorders are commonly seen in the paediatric intensive care unit (PICU). Severe metabolic acidosis is connected with adverse clinical outcomes. Of these, metabolic acidosis may occur due to a primary disease or as a result of secondary complications in patients with critical conditions. Metabolic acidosis is a pathological process defined by a decrease in the serum bicarbonate (HCO3–), an increase in the hydrogen ion (H+) concentration, and a secondary decrease in the partial pressure of carbon dioxide (PaCO2).1 It can be acute (occurring over minutes to days) or chronic (occurring over several weeks).1 Noor et al.2 found that approximately 44% of PICU admissions had metabolic acidosis. A similar study by Kim et al.3 reported that 60% of PICU admissions had metabolic acidosis. They concluded that regardless of underlying aetiology the corrected anion gap (AG) at PICU admission may predict mortality in children. Acute or chronic metabolic acidosis may affect cellular function adversely and result in increased morbidity and mortality.4,5
This article will review the approach and management of metabolic acidosis in children. The causes of metabolic acidosis will be addressed only briefly due to the constraints of space.
METHODS
The authors reviewed literature to identify data on the prevalence, manifestations, causes, outcomes, and management of metabolic acidosis in children. They searched the online databases (Ovid Medline, Embase, and PubMed) to identify relevant English language articles using combinations of (child OR children OR adolescent OR infant) AND (metabolic acidosis OR acidaemia). The authors also scanned the World Health Organization (WHO) website for further references. They screened abstracts of articles identified in this search strategy for relevance and obtained the full text of the electronic copies. They further scanned the cross references from each relevant article. Commercial search engines, including Google, were also used to check for any publications missed in the above searches. The authors also reviewed chapters on metabolic acidosis in the standard textbooks of paediatrics and medicine.
Definitions
Metabolic acidosis is defined as a pathologic process characterised by an increase in the H+ concentration and a reduction in the blood HCO3– concentration (<22 mmol/L). It can be acute or chronic.1,6
The severity of metabolic acidosis has been divided into three levels based on the systemic arterial blood pH: mild (pH: 7.30–7.36), moderate (pH: 7.20–7.29), and severe (pH: <7.20).
Acidaemia (as opposed to acidosis) is defined as a low arterial pH (<7.35), which can result from a metabolic acidosis, respiratory acidosis, or a combination.1,6
PATHOGENESIS OF METABOLIC ACIDOSIS
Under physiologic conditions, children produce 1–3 mmol/kg/day of non-volatile acid, predominantly sulfuric acid, derived from sulfur-containing amino acid metabolism. Acid-base balance is initially maintained by buffering of the acid load followed by urinary excretion. The HCO3– in the extracellular fluid and proteins, and phosphate in the cell provide the initial buffering,7,8 the net effect of which is a fall in serum HCO3– concentration. The renal excretion of the daily acid load then restores the serum HCO3– concentration to normal.
Two basic processes are involved, mediated by tubular H+ secretion: absorption of the filtered HCO3– and H+ secretion. These combine with either titratable acids, mainly phosphate (HPO4–+H+=H2PO4–) or ammonia (to form ammonium).7,8 In normal circumstances, the rates of titratable acid and ammonium excretion are roughly equal to the daily dietary acid load. The kidney compensates, primarily by increasing ammonium excretion when the acid load is increased.
There are three basic pathophysiologic mechanisms leading to metabolic acidosis, including1,9 an increase in acid concentration. This occurs either due to increased acid generation (endogenous production or exogenous ingestion/infusions) or decreased excretion of acid by the kidney (e.g., renal failure and distal or Type 1 renal tubular acidosis [RTA]). It also includes the loss of HCO3–, which occurs with a loss of serum HCO3– via the gastrointestinal tract (diarrhoea) or abnormal drainage from the small bowel/pancreas or from the kidneys (e.g., proximal or Type 2 RTA). The loss of serum bicarbonate is matched with a concomitant increase in serum chloride (hyperchloremic metabolic acidosis). Finally, it also includes the dilution of serum HCO3– concentrations, which pertains to a drop in serum HCO3– concentration due to intravascular fluid volume expansion with a large volume of non-HCO3–/acetate/lactate-containing solutions with a resultant rise in blood H+ concentrations. It is usually seen in children who receive parenteral fluids following trauma or in those undergoing surgery. Dilution-induced metabolic acidosis is generally mild and often occurs with clinical signs of fluid overload.
Adverse Effects of Metabolic Acidosis
Involvement of the cardiovascular system is most critical, though acute metabolic acidosis can affect a number of organ systems. The adverse effects of metabolic acidosis can be classified into those primarily occurring with acute metabolic acidosis and those with chronic metabolic acidosis.1,9
Clinical Features
There are no distinguishing symptoms of metabolic acidosis in children. Most features are non-specific, as detailed below.
Acute metabolic acidosis typically presents with symptoms related to the underlying condition and may also have signs/symptoms of compensatory respiratory alkalosis. Respiratory compensation can result in tachypnoea and deep respiration (e.g., Kussmaul breathing). The lack of an appropriate hyperventilatory response may be indicative of a significant underlying neurologic and/or respiratory disorder.9 Neurologic effects such as lethargy and confusion have been observed in patients with severe acute metabolic acidosis,1,10 as well as cardiac effects. Data from animal and tissue studies have shown myocardial depression and arrhythmias when the pH falls below 7.1.11
Chronic metabolic acidosis can be asymptomatic or present with multiple-system manifestations, depending on how long the underlying condition has lasted and how severe it has been. Findings in children with long-standing uncorrected metabolic acidosis (e.g., RTA) include poor growth and skeletal muscle wasting. Poor growth and skeletal muscle wasting are attributed to aberrant growth hormone secretion, resistance to insulin-like growth factors, and rickets due to bone abnormalities.12 These can result in poor growth and skeletal muscle wasting. It can also include rickets, where the release of calcium and phosphate from bone due to bone buffering of some of the excess H+ results in a decrease in bone mineral content and rickets.1,9,13 Another finding is nephrolithiasis and nephrocalcinosis. Increased mobilisation of calcium out of the bone results in an increased renal load of calcium leading to hypercalciuria. Additionally, hypocitraturia occurs in response to metabolic acidosis with enhanced proximal tubular citrate reabsorption. This limits the ability to reabsorb tubular calcium leading to nephrolithiasis and nephrocalcinosis.1,9,14
APPROACH TO METABOLIC ACIDOSIS
History and Physical Examination
History should be first evaluated in a child with metabolic acidosis. The clinical context will often point to the underlying disorder leading to metabolic acidosis. For example, metabolic acidosis in a child with severe diarrhoea suggests an acute HCO3– loss through the gastrointestinal tract, while recent-onset polyuria, polydipsia, weight loss, and hyperglycaemia suggest new-onset diabetes with ketoacidosis. A more detailed laboratory evaluation will be needed to determine severity, chronicity, and treatment options.1
Laboratory Evaluation
A diagnostic evaluation that includes serum electrolytes, blood glucose, renal function tests, serum lactic acid, serum AG (SAG), and urine AG usually suffice to specify the aetiology of the metabolic acidosis and direct therapy. In some cases, a blood gas measurement is needed to differentiate primary metabolic acidosis from compensated respiratory alkalosis.
Blood Gas
If metabolic acidosis is tentatively identified by a low total CO2 on an electrolyte panel, an arterial or venous blood gas sample may be needed to differentiate metabolic acidosis from compensated respiratory alkalosis. This eliminates the possibility of a primary respiratory alkalosis where the bicarbonate is decreased due to a compensatory renal response. If the patient has a simple metabolic acidosis, then the patient will be acidaemic with a pH of <7.35. If the patient has respiratory alkalosis with compensated metabolic acidosis, the patient is alkalemic with a pH >7.42.
An arterial blood gas also helps in evaluating for a co-existing mixed acid-base disturbance.1 As discussed above, acidaemia due to metabolic acidosis causes compensatory respiratory alkalosis. Documentation of the presence and magnitude of the respiratory compensation should be done. In uncomplicated metabolic acidosis, a predictable relationship exists between the change in (∆) PaCO2 and ∆ HCO3– concentration once a steady state is established. This can be derived using the Winter’s formula: predicted PaCo2=1.5 (HCO3–)+8±2.
A ∆ PaCO2 higher than expected indicates a co-existing respiratory alkalosis.1,15 On the other hand, if the ∆ PaCO2 is lower than expected, a co-existing respiratory acidosis is present.15 Once it has been established whether metabolic acidosis is present in isolation or in association with respiratory disturbances, the aetiology of metabolic acidosis should be investigated.
Anion Gap
Once metabolic acidosis has been confirmed, serum electrolyte values are used to calculate the SAG.1 The SAG is the difference between the serum concentrations of the primary measured cations namely sodium (Na+) and the measured anions, chloride (Cl−), and HCO3–.1,9
SAG=(Na+)–(C1–+HCO3–).
The normal SAG is 8–16 mEq/L (or mmol/L in SI units).9
The SAG, composed of unmeasured anions, is generally due to negatively charged plasma proteins. Negatively charged plasma proteins account for the normal AG, and, hence, normal values should be modified for patients with hypoalbuminaemia.16
Corrected SAG=(Na+)–([C1–+HCO3–)+2.5x[4.0-measured albumin g/dL).
The AG further divides patients into two groups: those with normal AG metabolic acidosis and those with increased AG metabolic acidosis. This allows an algorithmic approach to diagnosis. Figure 1 shows the algorithms for the initial approach to primary metabolic acidosis.
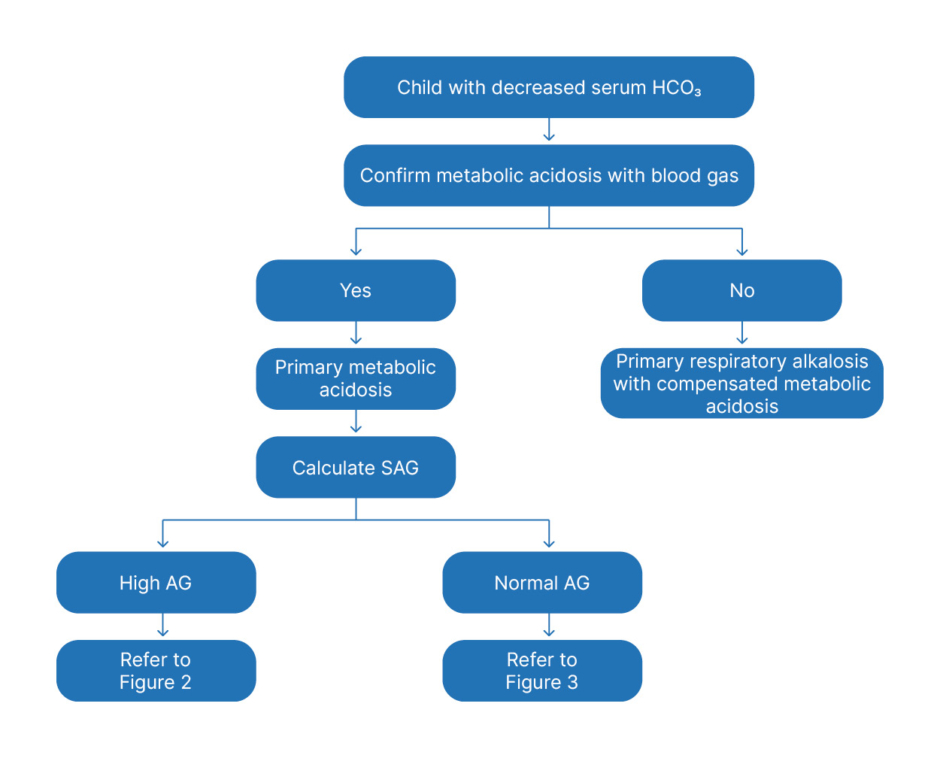
Figure 1: General approach to a child with metabolic acidosis.
AG: anion gap; SAG: serum anion gap; HCO3: bicarbonate.
Increased anion gap metabolic acidosis
Increased AG metabolic acidosis occurs when there is an increase in the number of both H+ and unmeasured anions.1,9 For example, in diabetic ketoacidosis (DKA), β-hydroxybutyrate and acetoacetate are the unmeasured anions. In lactic acidosis, lactic acid is composed of negatively charged lactate ions and positively charged H+. The H+ are buffered by serum HCO3– until reserves are depleted, at which point the residual H+ will lower the serum pH, while lactate anions will raise the AG. Results from the initial basic metabolic tests and the history and physical examination are typically helpful in determining the underlying cause and in some circumstances, guide further diagnostic evaluation. For example:
• Elevated blood glucose and a history of polyuria with or without weight loss, abdominal pain, and vomiting, point to DKA.
• Elevated blood urea nitrogen (BUN) and serum creatinine suggest uraemia and impaired renal acid excretion.
• Evidence of poor tissue perfusion (shock) with elevated lactic acid is seen in sepsis, cardiac failure, and severe hypoxia, as well as in severe crush injuries.
• Neurologic signs and symptoms: a history of severe hypotonia, seizures, developmental delay, or apnoea in a new-born infant may be suggestive of an inborn error of metabolism. Elevated lactic acid is observed in patients with mitochondrial disorders and organic acidurias. A serum ammonia level and a lactic acid and pyruvic acid ratio may be helpful in the differentiation of the inborn error of metabolism.
• History of accidental or intentional ingestion such as ethylene glycol, ethanol, or methanol ingestion (results in a high SAG and osmol gap); and elevated levels of iron, cyanide, carboxyhaemoglobin, salicylates, cocaine, or amphetamines, which are associated with high AG metabolic acidosis but with a normal osmol gap.
Osmolar Gap
The metabolites of certain toxic alcohols and glycols can generate a high AG metabolic acidosis. The accumulation of these alcohols, because of their low molecular weight, substantially elevates the serum osmolality and causes a disparity between the estimated and measured serum osmolality.17 Serum osmolality can be calculated using the following formula:
Calculated serum osmolality=2[Na+]+[Glucose]/ 18+[BUN]/2.8’ where [glucose] and [BUN] are measured in mg/dL.
Serum osmolality should be measured using a freezing point depression osmometer, not a vapour pressure osmometer, in conditions where the elevated osmol gap is generated by a volatile solute such as ethanol or methanol.17 The measured osmolality and the calculated osmolality should be similar (generally within approximately 6 mosmol/kg). An elevated serum osmol gap exists if the measured osmolality is more than 10 mosmol/kg greater than the calculated osmolality and suggests components such as methanol or ethylene glycol.1
Co-existence of Other Disorders
The presence of an elevated AG acidosis does not rule out a concomitant normal anion gap acidosis or a metabolic alkalosis. The delta ratio must be calculated to screen for mixed disorders and confirm that whatever acidaemia is present is completely explained by an elevated AG acidosis.18
Delta ratio=∆ AG/∆ HCO3
Delta ratio=measured anion gap–normal AG Normal HCO3 –-measured HCO3–
OR
Delta ratio=AG-12 24-[HCO3–]
Factors determining this relationship include the rates of urinary anion excretion and renal HCO3 generation, the spaces of distribution of the anions and protons, and the quantity of chloride-containing fluids infused.
In general, the Δ HCO3 is associated with an equivalent change in the Δ AG and this 1:1 relationship has been used to establish co-existing acid-base disorders, such as metabolic alkalosis or normal AG metabolic acidosis. A delta ratio of less than 1 suggests a concomitant normal AG metabolic acidosis, whereas a delta ratio of >1–2 suggests a concomitant metabolic alkalosis. The delta ratio is typically 1:1 in patients with keto-acidosis and often more than 1:1 (often as high as 1.6-1.8:1.0) in patients with lactic acidosis. It is usually less than 1:1 in patients with mixed non-AG metabolic acidosis and a high-AG metabolic acidosis in conditions where renal functions are preserved and the acid anion is readily excreted into the urine, for example with toluene ingestion and some cases of DKA.18
The algorithm for the evaluation of children with increased AG metabolic acidosis is given in Figure 2. A frequently used mnemonic to identify the more common causes of high AG metabolic acidosis in children is MUDPILES (where M is methanol; U is uraemia; D is DKA; P is paraldehyde; I is iron, isoniazid, and inborn metabolic errors; L ia lactic acid; E is ethylene glycol; and S is salicylates).9
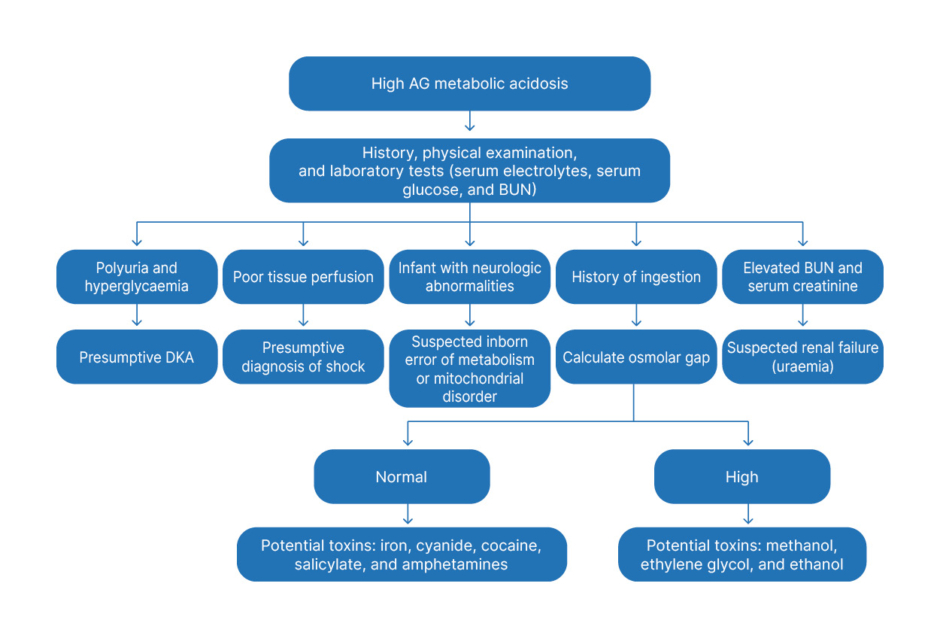
Figure 2: Approach to high anion gap metabolic acidosis in children.
AG: anion gap; BUN: blood urea nitrogen; DKA: diabetic ketoacidosis.
Normal Anion Gap Acidosis
Also referred as non-AG metabolic acidosis, this occurs with a loss of serum HCO3–. This loss of HCO3– is balanced by a concomitant rise in serum Cl– (hyperchloremic metabolic acidosis) to maintain electrical neutrality. Loss of serum HCO3– can occur via the gastrointestinal tract (diarrhoea) or abnormal drainage from the small bowel/pancreas or the kidneys (e.g., Type 2 or proximal RTA).1,9
If the aetiology of the normal AG remains unclear clinically, a urine AG may be useful. In the presence of metabolic acidosis, a positive value for urine AG is indicative of impaired ammonium excretion, such as is seen in distal (Type 1) and hypoaldosteronism (Type 4) RTA. Conversely, a negative value is consistent with intact urinary ammonium excretion as seen in children with metabolic acidosis due to proximal (Type 2) RTA and gastrointestinal losses.1,9,19
The urine anion gap can be determined using the equation: (Na+potassium [K])-Cl.1,9,19
The algorithm for the assessment of RTA is given in Figure 3.
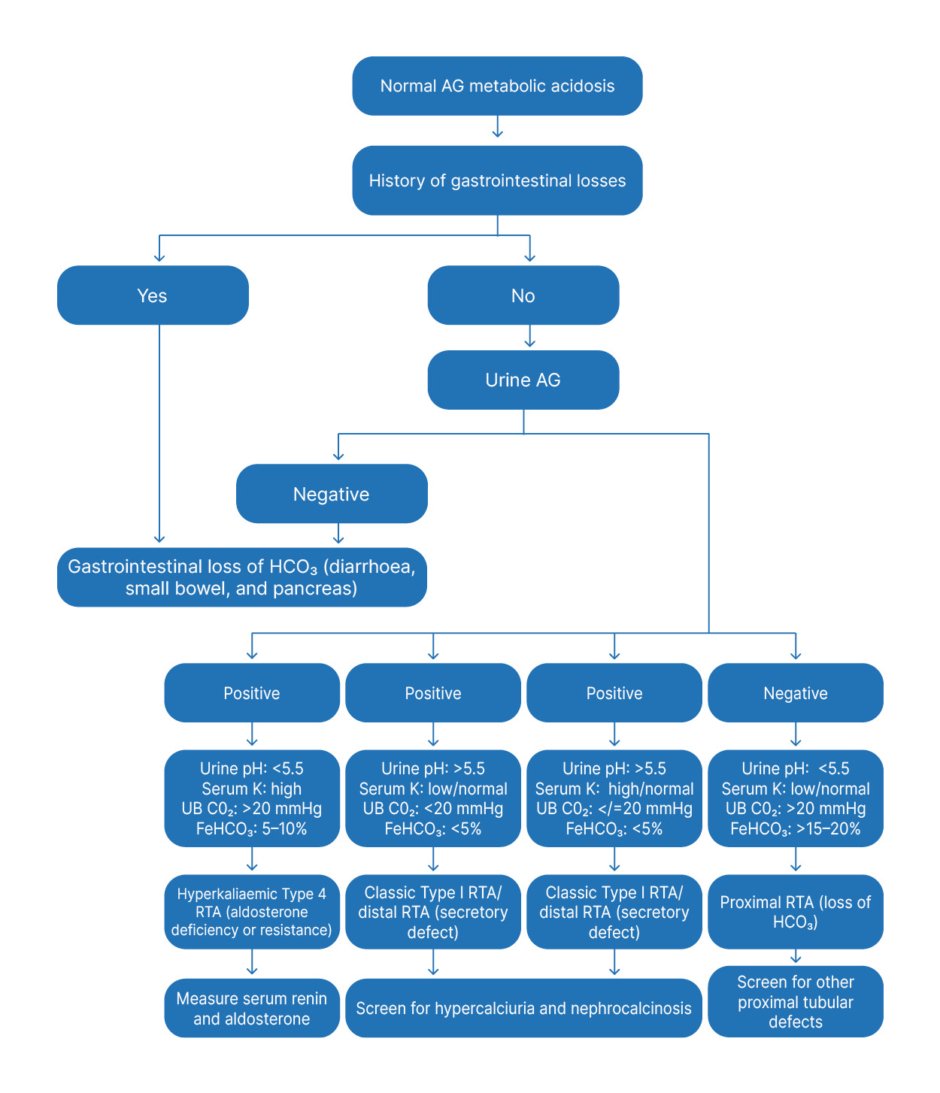
Figure 3: Approach to normal anion gap metabolic acidosis in children.
AG: anion gap; FeHCO3: fractional excretion of bicarbonate; HCO3: bicarbonate; K: potassium; RTA: renal tubular acidosis; UB CO2: urine-blood CO2 tension gradient.
MANAGEMENT
The primary focus of therapy for metabolic acidosis, both acute and chronic, is directed at reversing the underlying pathophysiologic process.1,9,19,20 Specific therapy of acidosis depends on whether metabolic acidosis is acute or chronic, and the severity of acidosis.
Acute Metabolic Acidosis
Mild metabolic acidosis is generally well tolerated but severe acidaemia can be life-threatening. In hypovolemic shock, restoring haemodynamic stability should be the priority. Similarly, delivering fluids and insulin to a patient in DKA remains the first-line therapy not only for the underlying condition, but also for the associated metabolic acidosis.
Intravenous bicarbonate therapy
When metabolic acidosis occurs due to loss of intracellular and extracellular buffers, it is compelling to administer NaHCO3 to shore up HCO3 stores. However, neither better clinical outcomes nor decreased mortality have been reported from the use of base therapy in cardiac arrest, lactic acidosis, DKA, and septic shock.21
Base therapy is disputed for several reasons. First, in conditions where HCO3 serves to buffer an increased acid load such as DKA or lactic acidosis, the use of HCO3 forms an anionic base known as potential HCO3, that can lead to overcorrection alkalosis.21,22 This in turn can result in dangerous degrees of hypokalaemia, hypophosphataemia, and ionised hypocalcaemia.22 Additionally, through the carbonic anhydrase-mediated reaction, HCO3 freely converts to CO2, which diffuses freely across cell membranes, and leads to a paradoxical intracellular acidosis and deranged cellular function.23 Besides, frequent NaHCO3 administration increases fluid overload and hypernatraemia risk, which can further worsen pulmonary oedema.21 In DKA, administration of base therapy increases the risk of cerebral oedema and delayed resolution of ketosis. Therefore, the risks of base therapy, in the conditions mentioned earlier, must be examined against the potential dangers of uncontrolled acidaemia.
Indications for sodium bicarbonate therapy
Severe metabolic acidosis affects the cardiovascular system most critically. A blood pH drop to below 7.1–7.2 is accompanied inevitably by a fall in cardiac output,24 a predisposition to ventricular arrythmias, and a resistance to the inotropic and vasoconstrictive effects of infused catecholamines.1,9 The use of intravenous (IV) NaHCO3 is reserved for the following cases:1,21
• IV HCO3should be used very cautiously if the pH is <7.1 with cardiovascular compromise. The aim is to raise the pH to 7.2. Since the PCO2 is expected to rise after NaHCO3, administration the patient should be adequately ventilated; severe hypokalaemia should be avoided with NaHCO3 therapy.
• IV HCO3 may be given when the blood pH is <7.2 in patients with impaired renal excretion such as those with RTA, acute kidney injury, and chronic kidney disease (CKD).
• IV HCO3 may be used in patients where urine alkalinisation is required therapeutically, as in patients with tumour lysis syndrome or rhabdomyolysis.
• In salicylate poisoning, base therapy increases renal clearance of salicylates and decreases the associated cognitive toxicity. Base therapy in salicylate poisoning is therefore recommended irrespective of the arterial pH.25
• Similarly, base therapy is also recommended to maintain a pH of 7.3 following the ingestion of ethylene glycol or methanol. Base therapy keeps the metabolites of these ingested substances in a charged form, limits their diffusion into end organs, and thereby reduces their toxic effects. Moreover, urinary alkalisation helps to increase renal clearance.25
Pre-administration considerations
Effect of NaHCO3 on the level of serum/plasma ionised calcium and compatibility with other IV medications should be taken into consideration prior to HCO3 therapy. Pre-treatment with calcium is advised if the corrected calcium is less than 8 mg/dL or ionised calcium is less than 1 mmol/L. This is important because an acute decrease in the ionised calcium concentration can cause cardiac dysrhythmias, seizures, and/or even tetany.9
Dose of intravenous bicarbonate
In the emergency setting, an 8.4% NaHCO3 solution is infused at a dose of 1 mEq/kg (maximum: 50 mEq), as an IV slow push. Blood gas, ionised calcium, and serum electrolytes are obtained after 15 min to determine therapy effectiveness and/or if there has been an adverse effect of bicarbonate therapy.9
In a non-emergency setting repletion, the dose of NaHCO3 is determined by the calculated estimated bicarbonate deficit using the following formula:9 estimated HCO3deficit=(target HCO3-current HCO3)xweight (kg)x0.4-0.5.
Half of the estimated HCO3 deficit is infused intravenously over 2–4 hours. The remaining half of the HCO3 deficit is infused over the following 6–24 hours. A longer course of infusion should be prescribed when the NaHCO3 deficit is large (>3 mEq/kg).
During and after repletion of the HCO3 deficit, blood gas, ionised calcium, and serum electrolytes are obtained to determine whether additional HCO3 therapy is required and to detect any adverse effect of HCO3 therapy.
When the patient can tolerate oral medications, maintenance administration should be changed to an oral alkali.
Chronic Metabolic Acidosis
Several studies in patients with chronic metabolic acidosis, with and without renal impairment, have shown that alkali therapy decreases the progression of bone disease, improves growth, reduces muscle degradation, and retards the progression of CKD.26
Oral Bicarbonate Therapy
Use of long-term base therapy is earmarked for children with disorders such as RTA and chronic renal failure. Oral HCO3 therapy is given in the form of NaHCO3, Na citrate, or K citrate. The choice of therapy depends on the underlying cause of chronic metabolic acidosis, availability and cost of the specific medication, and the experience of the prescribing clinician.9
Proximal/distal RTA can be effectively treated with NaHCO3/Na citrate. Citrate is actively metabolised to bicarbonate in the liver. The use of citrate solutions has been reported to ameliorate the harmful effects of chronic metabolic acidosis such as poor bone quality.26 For children with Type I and II RTA who are hypokalaemic, K citrate provides an added benefit. For those in renal failure, use of Na citrate prevents an added K load. Base therapy may exacerbate pre-existing hypertension and cause volume overload. Hence, care must be taken to maintain neutral sodium balance.
Treatment can be started at 1–2 mEq/kg/day of base in three to four divided doses. Some conditions like proximal RTA may require up to 20 mEq/kg/day of base for correction of acidosis. When alkali therapy is initiated, the smallest dose should be used and increased in a stepwise fashion until the targeted total CO2/HCO3 level is reached.
Mineralocorticoid therapy is indicated for the treatment of chronic metabolic acidosis in children with hyporeninemic states but should be used judiciously in those with antecedent hypertension.27 In such patients, diuretics or Na polystyrene sulfonate may be used for control of acidosis and hyperkalaemia.28
In patients with CKD on chronic haemodialysis (HD), dialysate with a high HCO3 concentration (approximately 40 mmol/L) usually suffices to correct metabolic acidosis.29 Similarly, in children on chronic ambulatory peritoneal dialysis (PD) a dialysate with a high base concentration is usually efficacious.29
Haemodialysis or Peritoneal Dialysis
Refractory acidosis is an indication for renal replacement therapy (HD or PD). The decision to perform dialysis may be dictated by concomitant electrolyte disturbances, especially hyperkalaemia. Besides providing base, HD is effective in removing toxins such as ethylene glycol or methanol. Continuous renal replacement therapy is superior to peritoneal dialysis or intermittent HD to finely control fluid and electrolyte derangements especially in haemodynamically unstable patients.30
PD using bicarbonate-buffered PD fluid may be required in children with seriously compromised liver functions and acute kidney injury.31 HCO3-based PD fluid may also be used for metabolic acidosis arising out of inborn errors of metabolism.31 The use of HCO3 as a buffer in the dialysate results in better correction of acidosis, lower lactate levels, and improved haemodynamic stability. HCO3-buffered dialysate cannot be stored; hence, it should be prepared just prior to use. Adding 260 mL of 5.0% dextrose and 40 mL of 8.4% NaHCO3 to 700 mL of normal saline gives a dialysate containing Na 147 mEq/L and HCO3 40 mEq/L).
OTHER TREATMENT MODALITIES
Tris-hydroxymethyl Aminomethane
Tris-hydroxymethyl aminomethane (THAM) is a reasonable alternative to HCO3 therapy in patients with acidosis and adequate renal function, particularly if associated with severe hypernatraemia and CO2 retention. THAM is a weak alkali that reduces arterial H+ without producing CO2. THAM, available as an IV formulation, can buffer acid by directly binding to protons without an associated increase in CO2.32,33 Another advantage of THAM is that it has the potential to optimise pH for cellular function since its diffusion intracellularly is slow.32 Adverse reactions of THAM include respiratory depression and hypoglycaemia due to insulin release, especially if infused rapidly. Though THAM has been tried in children with DKA and gastroenteritis, and in neonates with acute respiratory distress syndrome in the 1960s, there is limited published literature thereafter of its use in paediatrics or neonatology.32,33
Metabolic Acidosis as a Predictor of Mortality in Children
A variety of models exist for the prediction of mortality in patients of PICU, including the Pediatric Risk of Mortality (PRISM and PRISM III) and the Pediatric Index of Mortality (PIM and PIM 2) scores.34 These scores are a quantification of physiologic status using predetermined physiologic variables to facilitate accurate estimation of mortality risk. These scores have been validated in several studies, and help physicians to provide optimum patient management with the available resources. It is important to note that both the PRISM III and PIM 2 score use HCO3 levels and base excess as a variable for prediction of mortality. Kim et al.3 concluded in their study that an elevated corrected AG at admission was associated with higher mortality in PICU. They also suggested that corrected AG at admission may be used to predict mortality in PICU, regardless of underlying aetiology. Thus, metabolic acidosis plays an important role in determining the patient outcome and should be promptly addressed.
Effect of Metabolic Acidosis on the Quality of Life
Chronic metabolic acidosis can occur secondary to several conditions as mentioned above. It is important to study the quality of life in children affected with chronic conditions as it helps in providing a holistic, multidimensional, and comprehensive management.35,36 Some of these chronic conditions have associated metabolic acidosis. For example, studies on quality of life have been done in children suffering from CKD. Several of the clinical manifestations seen in CKD are due to underlying chronic metabolic acidosis.36-38 Ruidiaz-Gómez et al.36 did a meta-analysis on the impact of CKD on health-related quality of life in the paediatric population. They concluded that paediatric patients with CKD have lower health-related quality of life than healthy patients, specifically in the school, physical, emotional, and social dimensions. Amongst the affected dimensions, the most affected was the school attendance due to frequent visits to the doctor and the symptoms of the disease. Complications of the disease also reduced the opportunities for social interaction with peers and to carry on recreational activities, thereby affected the social dimension.
CONCLUSION
Metabolic acidosis is a common disorder in children and may present acutely or chronically. Diagnosis requires a methodical approach starting with the history and physical examination followed by laboratory investigations. The SAG plays a crucial role in differentiating the different causes of metabolic acidosis. Severe and prolonged metabolic acidosis has detrimental effects on cellular function and is related to increased morbidity and mortality. The main aim of treatment in metabolic acidosis is to reverse the primary pathophysiology. In acute metabolic acidosis, NaHCO3 therapy is not beneficial due to potential complications and is reserved for specific situations. Base therapy is used in chronic metabolic acidosis where it ameliorates many of its untoward effects.