Abstract
Pancreatic cancer (PC) still represents an unresolved therapeutic challenge due to the associated poor prognosis and the lack of responsiveness to current treatments. Surgery, followed by adjuvant therapy, is the only potentially curative treatment for PC; however, only 20% of PC patients have a potential resectable disease at diagnosis and the overall 5-year survival rate does not exceed 20%. In this context, better, more effective strategies are needed. Immunotherapy is an interesting approach for cancer treatments, but increasing evidence testifies that the immune system plays contrasting roles in both tumour elimination and tumour progression. In particular, PC is considered relatively immune resistant due to the characteristic fibrosis, the presence of immunosuppressive cells, and the compact extracellular matrix that defines the tumour microenvironment and allows for the growth of cancer cells. Despite this, there is evidence that PC cells are able to induce an anti-tumour immune response that can impact the disease course. More recently, it has become clear that PC activates both the anti-cancer immune response and the immunosuppressive effects of the immune system; therefore, for the immune-therapeutic strategies to be effective, they should involve not only the stimulation of the immune system but also the control of the immunosuppressive milieu. In this review, we discuss the dual role of immune cells in the onset and progression of PC.
INTRODUCTION
Pancreatic cancer (PC) still represents an unresolved therapeutic challenge due to the associated poor prognosis and the lack of responsiveness to current treatments. Surgery, followed by adjuvant therapy, is the only potentially curative treatment for PC, but only 20% of PC patients have a likely resectable disease at diagnosis and the overall 5-year survival rate does not exceed 20%.1 In this context, better, more effective strategies are needed. Several published data confirm that innate and adaptive immune cells, as well as effector molecules and pathways, can perform tumour suppressive mechanisms. However, we know that the immune system can also favour tumour progression. The Janus-faced role of the immune system is well-described by the dynamic process termed ‘cancer immunoediting’ (Figure 1).2
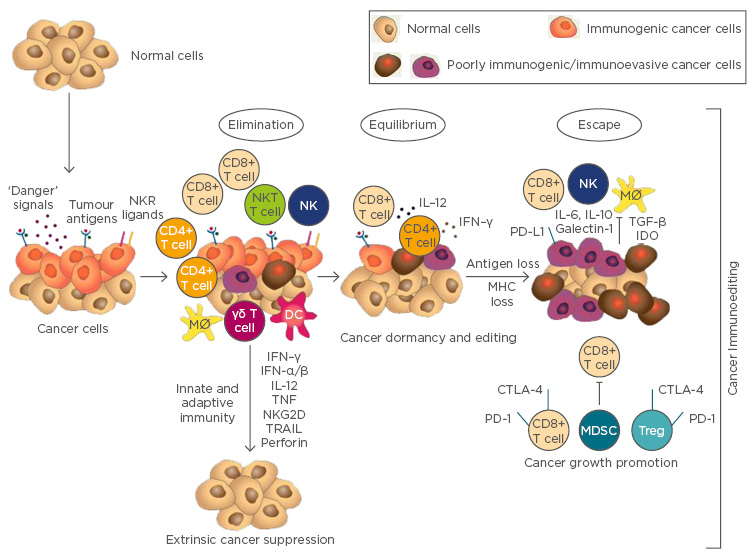
Figure 1: The three phases of the cancer immunoediting theory: Elimination, Equilibrium, and Escape.
Elimination: The effector immune cells successfully eradicate the developing tumour. Equilibrium: The tumour cells and the host immune system are in balance; the adaptive immune system controls tumour growth, although shaping tumour immunogenicity. Escape: The tumour overcomes the immune system and becomes a clinically evident disease.
CTL: cytotoxic lymphocytes; DC: dendritic cell; IFN: interferon; IL: interleukin; Mø: macrophage; MDSC: myeloid-derived suppressor cell; MHC: major histocompatibility complex; NK: natural killer cell; NKT: natural killer T cell; PD-1: programmed cell death 1: TNF: tumournecrosis factor; Treg: T regulatory cell; TGF: transforming growth factor.
Anti-tumour immune responses are carried out by both innate and adaptive immune system components, such as immune cells, cell surface molecules, costimulatory receptors, ligands, and cytokines. The innate immune cells principally involved in the fight against cancer are granulocytes, macrophages, natural killer (NK) cells, and dendritic cells (DC). These cells represent the first line of defence against invading and transformed cells, even though cancer cells can easily escape their surveillance. Other than this initial defence, the adaptive arm of the immune system, mainly represented by T and B lymphocytes, is the most important force against cancer and has the ability to recognise cells expressing foreign antigen-like neoplastic cells. Moreover, immunosuppressive cytokines and regulatory cell populations, including regulatory T cells (Treg) and tumour associated macrophages (TAM), can favour tumour growth. In this context, the action of the host’s immune response against tumours can be demonstrated by the presence of immune components in situ. Analysis of the density, location, and functional properties of all immune cell types that may be found in pancreatic tumour tissue has allowed the identification of the components that exert an anti-tumour or a tumour-supportive role in patients (Figure 2).
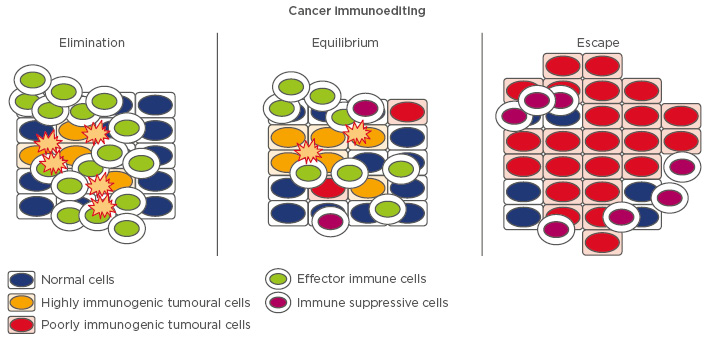
Figure 2: Diagram of cell types that interact with cancer cells in the tumour microenvironment.
Immune cells that exert an anti-pancreatic cancer effect are CD8+ cytotoxic lymphocytes, natural killer T cells, CD4+ T helper (Th1, Th9, Th17), natural killer cells, and dendritic cells. Cells with a pro-pancreatic cancer role are Th2, Th22, T regulatory cells, myloid-derived suppressor cells, tumour-associated macrophages, cancer-associated fibroblasts, and mast cells.
From an immunological perspective, PC is considered an incredibly peculiar cancer type. It is well known that inflammation drives PC development and progression; >90% of all pancreatic adenocarcinomas carry an activating mutation in the RAS oncogene, which drives an inflammatory programme.3 In addition, chronic inflammation (pancreatitis) is a documented risk factor for PC development.4 Although often characterised by a marked leukocyte infiltration,4 levels of intratumoural effector immune cells are limited in PC compared to other cancers.5,6 The lack of effective immunity accompanies a massive infiltration of immune suppressive leukocytes and the presence of tumour supportive immune cells. Usually, the PC stroma encompasses the majority of the tumour mass and consists of a dynamic assortment of extracellular matrix components and non-neoplastic cells, including fibroblasts, vascular cells, and immune cells. In particular, the expansion of the tumour is associated with a substantial desmoplastic stromal reaction that changes the normal pancreatic architecture into fibrotic tissue; this fibrotic tissue primarily represents a barrier for the immune system but also interacts with neoplastic cells, favouring its development.7
The PC microenvironment develops an immunosuppressive phenotype, as demonstrated by the large infiltration of Treg and myeloid-derived suppressor cells (MDSC) present at the early stage of the disease. In advanced PC, T lymphocytes (CD4+ and CD8+) are rarely found in the tumour tissue.8 In all stages of the disease, a strong inverse correlation between MDSC and CD8+ T cells persists, suggesting that MDSC are a mediator of tumour immune suppression.8 Nevertheless, PC is able to activate an anti-tumour immune response; firstly, the accumulation of CD8+ T cells in PC correlates with survival of patients9 and, secondly, the PC cells can express tumour-associated antigens10,11 that are immunogenic and able to elicit a specific immune response. For example, PC patients can show intratumoural and circulating alpha-enolase-specific T cells, and the presence of anti-alpha-enolase antibodies correlates with a significantly better clinical outcome in advanced patients treated with standard chemotherapy.11-13 For these reasons, despite both clinical and animal models showing strong evidence for inhibition of immune function in PC, patients can be candidates for immunotherapies, involving strategies that use stimulation of the immune system in order to control the tumour immune suppressive microenvironment.
ANTI-TUMOUR IMMUNE CELLS IN PANCREATIC CANCER
Effector T Cells
CD3+ T cells recognise antigens of cancer cells and are divided into either CD8+ cytotoxic lymphocytes (CTL) or CD4+ T helper (Th) cells that recognise peptides presented by the major histocompatibility complex (MHC)-I or MHC-II, respectively. The Th cells are divided into various subsets, including interferon (IFN)-γ and tumour necrosis factor (TNF)-α producing cells (Th1), interleukin (IL)-4, IL-5, and IL-13 expressing cells (Th2), T follicular helper cells (Tfh), IL-17 expressing cells (Th17), IL-9+ (Th9), IL-22 producing cells (Th22), and Treg. In addition, CTL subsets, including Tc1, Tc2, Tc17, and regulatory CD8+ T cells, have been defined. All these T lymphocyte subsets have been investigated for their implications in cancer development and anti-tumour immunity; due to their ability to produce IFN-γ and directly kill target cells, CTL are the critical mediators of the anti-tumour response.2
CD3+ T infiltrating lymphocytes (TIL) have been detected in human and mouse PC tissue specimens.8,14 Interestingly, the study of TIL distribution, density, and function in the tumour microenvironment reveals their anti or pro-tumour activity.6 In the case of PC, TIL do not usually reach the tumour cells in a consistent number, remaining confined in the peritumoural tissue.14 One explanation for poor CTL infiltration may be the lack of neoantigen expressed by PC cells.15 Moreover, it can be due to the effects of the stroma and suppressor immune cells.16
The presence of TIL in PC was reported for the first time by Ademmer et al.17 They documented that lymphocytes were most often confined as agglomerates in the fibrotic interstitial tissue, while rare cells could be found among the epithelial neoplastic cells. The percentage of lymphocytes was changeable between samples; however, there was a predominance of T cells with a CD45RO+ memory phenotype. Among the TIL, the memory CTL exert the major antitumour effects and their frequency in resected PC was found to correlate with survival.18
The role of Th cells in tumours is more complicated than the role of CTL because their protective or supporting role towards cancer cells depends on their functional profile. For example, Th2 cells take part in tumour tolerance,6 while Th1 cells antagonise tumour growth through the production of IL-2 and IFN-γ, required for the activation and proliferation of CTL activity.19,20 Overall, memory T cells and/or T cells with a Th1 phenotype are associated with a better prognosis.21
The role of Th17 cells in tumourigenesis has not been clarified. Both animal models and clinical studies have suggested functions for Th17 cells (and related cytokines) in tumour development. He et al.22 detected Th17 cells in pancreatic tumour tissues and showed that the frequency of Th17 cells was significantly higher in the lymphocytes infiltrating the PC than the adjacent tissue; in addition, the number of Th17 cells was associated with tumour stages.22 We have recently demonstrated that ENO1-specific Th17 cells have a specific anti-cancer effector function in PC patients, and that there are decreased levels of these cells in cancer compared to healthy mucosa.12
The recently discovered subset of Th lymphocytes, named Th9 cells, secrete IL-9 together with IL-21 and play a role in several inflammatory disorders. These cells arise from reprogrammed Th2 cells upon stimulation with IL-4 and transforming growth factor (TGF)-β.23 Th9 cells feature potent anticancer properties,24,25 but currently there are no data on their role in PC. Natural killer T (NKT) cells are a small subpopulation of T lymphocytes with antigen-specific T cell receptors (TCR) that recognise both self antigens and foreign antigens, providing a mechanism to identify lipid antigens that are not detected by CD4+ and CD8+ T lymphocytes. In cancer, these cells were initially considered to exert a defensive role, but in recent studies they have been found to also inhibit anti-tumour responses. It is now more clear that this dual role depends on the presence of two different subpopulations of NKT cells with distinguished functions: type I NKT cells with an invariant TCR and type II NKT cells with more variable TCR. type I NKT cells support tumour immunity through the production of IFN-γ, activating NK cells, CTL cells, and DC to produce IL-12. Conversely, Type II NKT cells, distinguished by differing TCR (recognising lipids presented by CD1d), principally obstruct tumour immunity.26 Statistically significant lower numbers of peripheral blood NKT cells were found in patients with a variety of cancers compared to healthy subjects.27 Nagaraj et al.28 attempted to enhance the anti-tumour effect against PC by supplementary triggering of NKT cells in vivo; they established a significant expansion of IFN-γ-producing NKT cells correlates with reduced tumour growth.
Natural Killer Cells
NK cells are innate lymphocytes known as the first-line of defence against infections and neoplasia due to their ability to exert direct cellular cytotoxicity without prior sensitisation and to secrete immunostimulatory cytokines like IFN-γ. Their ability to spontaneously kill tumour cells and to activate other immune cells highlights the importance of their role in fighting primary tumours and metastases. Apart from conventional NK cells, there is a range of tissue-resident NK cells with considerable differences in terms of their origin, development, and/or function.29 The discovery of the diversity among NK cells, and the newly arising innate lymphoid cells (ILC), in general led to a newly proposed nomenclature; thus, conventional NK cells are classed as belonging to the Group 1 ILC, distinguished from ILC2, ILC3, and other non-NK cell ILC1 subsets by their dependence on IL-15 and their intrinsic cytotoxic capacity.30 The scanning mechanism of NK cells is what allows them to maintain surveillance of tumour cells and virus-infected cells.
Through MHC Class I loss, a common event in PC,31 PC cells become the target of NK cells, even if they can escape from this control system. Indeed, activating receptors, such as NKG2D, are reduced on the surface of NK cells in patients with PC and these lower levels are associated with advanced disease.32 Higher absolute levels of NK cells in the circulation were associated with a better prognosis in a small set of 13 patients with PC,33 indicating that the immune system, through NK cells, still exerts some control on cancer growth despite disease progression.
Dendritic Cells
DC are important for immune surveillance and play a key role in cancer immunosurveillance. Indeed, induction of an effective anti-tumour response requires the active participation of host antigen presenting cells, such as DC, responsible for the presentation of tumour-specific antigens with the ability to elicit primary immune responses. DC are divided into two subsets: myeloid CD11c+ DC (DCs1) and lymphoid CD11c− DC (DCs2), which have been shown to regulate immune responses via the polarisation of Th1, Th2, or even Th3 differentiation under the influence of cytokines produced by themselves. For this reason, some biological response modifiers used in anticancer therapy upregulate the activity of DCs1, but not DCs2 activity.34 In patients with PC, DC presence is associated with a better prognosis,35 even if their number and function, in particular of DCs1, is decreased and defective, limiting their ability to present foreign antigens to T cells.
IMMUNE CELLS WITH PRO-PANCREATIC CANCER ACTIVITY
Tumour-Supportive T Lymphocytes
Treg are a subset of T lymphocytes, characterised by the expression of the transcription factor forkhead box protein P3 and by the production of cytokines, such as TGF-β and IL-10. They modulate the immune system, maintain tolerance, and prevent autoimmune disease. In terms of immunosuppressive activity, Treg are able to inhibit anti-tumour immune responses and are a negative prognostic indicator in various tumours. Treg were found in greater numbers in PC tissue than in non-tumoural pancreatic stroma,12,36 and higher levels of Treg correlated with less differentiated tumours.37 Moreover, Treg correlate with metastasis and tumour grade, and negatively correlate with patient survival.5,36,37
Immunohistochemical assays on PC tissues show the presence of both Th1 and Th2 cell subpopulations, with the prevalence of the Th2 subset.38 A recent study39 reported that the Th2/Th1 ratio is an independent predictor of disease-free and overall survival in PC patients, suggesting the implication of Th2 in cancer promotion. The pro-tumoural effect of Th2 polarisation depends on IL-4 production that, besides reducing Th1 polarisation, has a direct immunosuppressive effect on CD8+ T cells.40
Th22 cells, a T helper subpopulation producing IL-22, were first described in patients with inflammatory disease but have also been identified in many tumours.41,42 Even if the role of Th22 lymphocytes in the cancer immune response is ambiguous, IL-22 seems to play a pro-tumoural role in gastrointestinal tumours.42 In PC, the intratumoural IL-22 levels and frequency of Th22 cells are elevated compared with the peripheral blood of patients and healthy donors.43 Moreover, the expression of both IL-22 and IL-22R1 is elevated in tissue sections of PC.44 Recently, we observed that IL-22-producing T cells were significantly increased in PC tissue and that this increase was positively correlated with tumour, node, and metastasis (TNM) staging and poorer patient survival.41 We also demonstrated that IL-22 production by intratumoural Th1/Th22 cells during PC progression may therefore neutralise the anti-tumour effect of Th1-polarised T cells, protecting the cancer cells by the proapoptotic effect of IFN-γ. Moreover, the percentage of peripheral blood Th22 cells in PC patients was significantly higher compared to age-matched healthy donors, demonstrating that monitoring circulating Th22 levels could be a good diagnostic parameter and blocking IL–22 signalling may represent a viable method for innovative anti–PC therapies.
Tumour-Associated Macrophages
Frequently, cancers are infiltrated by TAM, classically divided into two subsets, M1 and M2. M1 macrophages possess proinflammatory and tumouricidal capabilities, while M2 are specialised to suppress inflammation and repair damaged tissues. TAM seem crucial in mediating PC immune escape. Kurahara et al.45 suggested that increased M2-type infiltration might support the lymph-angiogenesis and lymphatic metastatic spread in PC; moreover, PC patients that display a high TAM infiltration had a significantly poorer prognosis. In accordance, Ino et al.5 showed that high CD163+ and CD204+ cell infiltration correlated with a reduced disease-free and overall survival in 212 PC patients. Recently, it has been demonstrated that the depletion of a specific extratumoural macrophage population can enhance CD8+ T-cell tumour infiltration in response to CD40 agonist immunotherapy46 and, furthermore, Rosati et al.47 showed that the blocking of macrophage activation leads to diminishing primary tumour growth and metastasis.
Myeloid-Derived Suppressor Cells
MDSC are a heterogeneous population of cells defined by their myeloid origin, immature state, and ability to potently suppress T cell responses. Where MDSC are missing in the normal pancreas, they are quickly recruited to the PC stroma, where they can represent >60% of the infiltrating leukocytes.48 In a spontaneous PC mouse model, Zhao et al.49 have shown that, in the pre-malignant lesion stage, MDSC count is increased in the lymph nodes, blood, and pancreas, and that this increment becomes greater upon tumour development. In vitro, MDSC were capable of suppressing T lymphocyte responses. More recently, MDSC infiltration was correlated with decreased levels of CTL and T helper cells and increased levels of Treg in the blood of mice after subcutaneous injection of the non-metastatic PC cell line, Pan02. Furthermore, MDSC were able to suppress CTL in vitro and induce initial cancer growth.48 Indeed, in the KrasLSL.G12D/+; p53R172H/+; PdxCretg/+ (KPC) model of metastatic PC, MDSC correlated with cancer cells and metastases, suppressed T cell proliferation, and expressed high levels of arginase and nitrite upon stimulation.50 Gabitass et al.51 showed that in 46 PC patients, MDSC levels correlated with a Th2 skewing for cytokine production, in particular for IL-13 and, moreover, a high concentration of MDSC in the peripheral blood was associated with poor outcomes.
Mast Cells
The role of mast cells (MC), a type of granulocyte derived from the myeloid stem cell, in cancer is not well-defined,18 but it is known that MC are a consistent component of the tumour microenvironment in different human cancers and, depending on the tumour, MC counts have been associated with either favourable or poor prognosis. In patients with PC, MC numbers were significantly increased in tumour tissue compared to the non-tumoural pancreas and their count correlated with lymph nodes’ metastases and intratumour microvessel density; furthermore, patients with a low count of infiltrating MC tended to survive longer than those with elevated numbers.52 More recent research,53 conducted on 53 PC patients, showed that a massive MC infiltration was present in higher grade tumours and recurrence-free and disease-specific survival was worse in patients with high numbers of MC than those with a lower MC count. In another study, comprising 67 PC tissue samples, high infiltration of MC was confirmed as a negative predictive marker of patient survival.54
CONCLUSION
Immunotherapy appears to be a promising treatment for cancer, including PC.55,56 Currently, many clinical trials for PC treatment are ongoing, based on synthetic, cellular-based, autologous and allogeneic vaccines, adoptive T-cell transfer, and combination therapies. However, many of these approaches, designed to prompt or increase the anti-tumoural immune response, failed due to the immunosuppressive microenvironment that characterises PC. Indeed, in evaluating the opportunity of immune-based therapies, it is crucial to remember that from an early stage in PC progression, the capacity of the immune system to recognise and eliminate tumoural cells is impaired, due to the ability of PC cells to affect both arms of the immune response (immune activation and suppression) and taking into account that unspecified immunotherapeutic approaches also led to the stimulation of immune suppression. In conclusion, future concepts of PC immunotherapy should be designed based on the elimination of immune suppression. In order to perform an effective therapeutic strategy, it is necessary to increase the density of intratumoural effector T cells, decrease or inhibit suppressor immune cells and receptors, and understand the role of stromal reaction and its interaction with PC immune microenvironment.