Abstract
Percutaneous nephrostomy insertion is a technique performed by an interventional radiologist or a urologist for an acutely or long-standing obstructed urinary tract. Mastering the technique involves overcoming a steep learning curve. Various methods of training have been developed over the years to facilitate learning. These vary from simple physical models, such as biological or non-biological practice phantoms, to more sophisticated virtual reality sets, which allow for a more lifelike learning environment by replicating factors such as kidney movement caused by breathing. The authors discuss the pros and cons of different practice models and the challenges that trainees face on their journey to becoming competent at performing nephrostomies. They also propose their recommendations based on the experience of trainees in their institution.
Key Points
1. Percutaneous nephrostomy insertion is a highly effective, low-risk technique performed by interventional radiologists or urologists. Different methods of training exist, using both in vivo and ex vivo techniques.2. Hybrid ex vivo models of training, used in conjunction with traditional teaching, can achieve greater competence and confidence in trainees in a shorter space of time than could ever be achieved with conventional in vivo training.
3. Ex vivo training tools can not only increase early exposure to the steps of percutaneous nephrostomy in a safe, risk-free environment, but can be used to train greater numbers of trainees than in vivo cases can.
INTRODUCTION
Urinary tract obstruction is a common acute presentation to secondary care. Relief of upper urinary tract obstruction is usually performed in acute cases, or in chronic obstruction where treatment of the causative process is not immediately possible. Common benign causes of an acutely obstructed renal tract are renal calculi, pelvic ureteric junction obstruction, and retroperitoneal inflammatory or fibrotic processes. Chronic obstruction may be due to pelvic malignancy, pregnancy, or urothelial strictures.
Percutaneous nephrostomy (PCN) is a commonly performed procedure for decompression of an obstructed urinary system. First described in 1955, it has since been widely adapted with a good success rate, low complication rate, and without the need for general anaesthesia.1
Formal curriculum advice regarding the skill of percutaneous renal access for both radiology and urology trainees is widely variable, and currently implemented restricted working patterns have a limiting impact on surgical training opportunities.2 The authors discuss the challenges faced by trainees in gaining exposure to PCN insertion, and detail some of the options available to enhance training.
PERCUTANEOUS NEPHROSTOMY: WHO DOES IT Affect AND HOW?
In the UK, PCN is a core competency, as recognised by the Royal College of Radiologists (RCR), for higher interventional radiology trainees. In most cases, percutaneous renal access is obtained by radiologists. In comparison, in Europe, both radiologists and urologists are trained in percutaneous access. Increased exposure and training in both ultrasound skills and PCN in the core urology curriculum would undoubtedly alleviate the pressure on interventional radiologists, as well as provide urologists with an ability to perform endourological procedures independently.
Access to the renal system is achieved by image guidance, with multiple modalities available. Initial needle puncture is commonly performed under ultrasound guidance, and subsequent intervention to the urinary tract can be carried out under ultrasound or fluoroscopic guidance. Fluoroscopy enables the operator to visualise the upper renal tract in real-time, with an option to image from different angles, allowing for easy assessment of the position of interventional wires and catheters used during the procedure. PCN can be performed in conjunction with the insertion of J stents, and cases are usually collaborated and discussed between radiologists and urologists with regard to the preferred order of intervention. It is also the initial step that allows access to the upper renal tract in common urological procedures, such as percutaneous lithotripsy.
COMPLICATIONS OF PERCUTANEOUS NEPHROSTOMY
PCN is a highly effective and relatively low-risk procedure. A study describing the safety profile of PCNs performed by radiologists conducted on large numbers of patients reported success rates as high as 98.0%, with a relatively low complication rate of 6.5%.3 Similarly, high success rates and low complication rates have been reported in various countries, including Sweden, Pakistan, and the UK.4-6
Reviews of patient outcomes after PCN tube insertion done by urologists are equally favourable. In a retrospective analysis of 650 percutaneous nephrostomies carried out over a 10-year period by both urology consultants and registrars, Skolarikos et al.7 found similarly high technical success rates of 96% and 93%. Major complication risks were also found to be low: 3.6% for registrars and 3.1% for consultants.
Potential complications are rare, albeit possible. These are frequently classed as early (such as sepsis, retroperitoneal haematoma, bleeding, solid organ injury, and urinoma) or late (for example, catheter blockage or dislodgement). Reported complication rates for radiologists as well as urologists are relatively low, without major discrepancies between the two groups.5
Review of data from the contemporary UK setting yields congruent findings. Armitage et al.8 conducted the first UK national comparison of outcomes of percutaneous nephrolithotomy (PCNL), obtained by urologists versus interventional radiologists. They found that of the 5,211 procedures that were done between 2009 and 2015, 66.3% were carried out by interventional radiologists. They found no major patient outcome differences between the two groups, and favourable results were achieved regardless of whether the procedure was done by a urologist or an interventional radiologist. Interestingly, the authors noted some differences in practice between the two groups, such as the much higher use of ultrasonography by radiologists, and a higher rate of supine punctures performed by urologists.
Studies have shown that there is a correlation between the operator’s experience level and the post-procedure complication rate. A 10-year review found that inexperienced operators (those who had performed less than 20 PCNs, with a mean of 5.6) were found to have a 17% complication risk. Bleeding and urinary leaks were the most common problems. Of the patients who had PCN performed by experienced operators (at least 20 prior procedures, with a mean of 178 cases), only one required a further intervention for their complication, whereas the less experienced operator group had 10 patients who required a repeat procedure.9
METHODS OF TRAINING
In the past, experiential learning underpinned surgical training. The ability to perform procedures under close supervision, with feedback from senior clinicians, was the only available method for trainees to progress in training. Factors such as increasing demand, pressures on operating and interventional rooms, and fear of litigation make this approach less favoured nowadays.10 Learning of any new procedural skill always starts with the acquisition of knowledge and the theory that underpins it. Equally important is the experience; in the hands of an inexperienced operator, PCN is a potentially risky task, with the potential for complications. As such, there is a real need to be able to simulate PCN to allow trainees to practice. Many such methods for training already exist. Here, the authors discuss the pros and cons of methods put forward as a means of training junior radiologists and urologists in PCN and renal access.
Simulation-Based Training
Simulation has been a common method of training. It is already a well-established method of training in aviation, where, understandably, training in vivo would be high-risk. Methods vary from simple physical models to more sophisticated virtual reality (VR) sets, where computerised technology is used to mimic variable anatomical and real-life factors, such as respiratory movement of the kidney. They can also provide real-time feedback by measuring predetermined factors, such as the dose of radiation used during the simulation.11
Simulation allows trainees to repetitively practice a skill in a pressure-free environment. It allows theoretical knowledge obtained outside of the classroom to be applied safely into practice, as well as for mistakes to be made in a controlled, safe environment, where direct feedback can be obtained. Based on the fidelity, simulators can be classified into two categories: low-fidelity (suturing pads) and high-fidelity simulators (Harvey cardiology manikin). Simulator fidelity must be considered when developing simulation programs. Rudolph et al.12 suggested that three features of simulation fidelity influence the overall experience of the learner. These are physical fidelity (physical attributes of the equipment and environment), conceptual fidelity (the actions and events make sense), and finally, experiential and emotional fidelity (the holistic experience, including the emotions and cognitive states of the participants). The impact of simulation fidelity on learning outcomes may vary, based on the trainees and training goals. It is possible that a low-fidelity model would be acceptable to accomplish modest improvements for novices learning the task, but not mastery. It is, therefore, important to consider both fidelity and instructional design before planning a training course.
Benchtop Models
More accessible benchtop models exist and these can be both biological (often consisting of a porcine kidney within a mould) or non-biological. The main pros of benchtop models over wet-lab models are the ease of set-up, and the ability to practice repetitively (Figure 1A). These benchtop models are also cheaper than more high-tech simulators.13
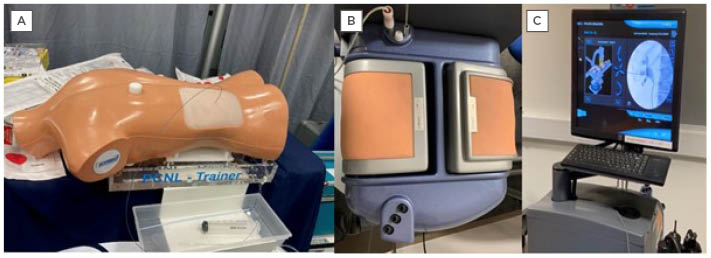
Figure 1: An example of a commercially available benchtop model available for percutaneous nephrolithotomy training.
A) Samed PCNL Training Device LS40 bench-top model; B) PERC Mentor™️ (3D Systems [formerly Simbionix USA Corp.,], Rock Hill, South Carolina, USA); flank pad; and C) display monitor.
Phantom Models
Benchtop phantom models are another modality of training used in other factions of surgical and radiological training, from basic suturing skills to more advanced vascular interventions. A study conducted at the Department of Urology, University of California, San Francisco, California, USA, found that confidence with percutaneous renal access increased after the use of a phantom model for training, alongside direct senior clinician feedback. Fifteen urology trainees were divided into three groups, and underwent timed trials on a phantom model for percutaneous renal access. Group 1 was given access to the phantom model prior to the time trial; Group 2 was given a teaching session on renal access by a senior clinician, followed by access to the phantom; and Group 3 was given a teaching session and access to the phantom for practice, in addition to individual feedback from a senior clinician whilst using the phantom prior to the time trial. The results of this study demonstrated that urology trainees in Group 3 demonstrated the greatest improvement in technical skills assessed, including the number of attempts to gain renal access, the accuracy of needle puncture, and time to needle placement.14 Although nephrostomy and renal access phantoms have their benefits in training, the cost can limit availability across training schemes. Rock et al.15 submitted an easily reproducible and cheap gelatin-based phantom model to the British Society of Radiology (BIR) in 2010. They suggested that a hydronephrotic collecting system could be replicated by a fluid-filled and tied-off disposable vinyl glove. Gelatin surrounding this simulated kidney was used to replicate the renal parenchyma. This was then inserted into a plastic bottle split mould, and refrigerated to simulate a hydronephrotic kidney. It was subsequently inserted longitudinally into a gelatin-filled plastic box, and cooled again to create the final phantom model, in which ultrasound-guided PCN could be simulated, including the ability to aspirate fluid to confirm needle placement. Although not statistically supported, this is an example of how benchtop phantoms can be easily and cheaply reproducible, to allow ex vivo training opportunities.15
The SimPORTAL C-arm Trainer contains a flank model with an anatomically accurate cast of the pelvicalyceal system and the ureter, with an overlay of ribs for needle puncture, and a mini C-arm for fluoroscopic simulation, with two mounted video cameras. Approximately 92.8% of the 14 enrolled participants considered the SimPORTAL C-arm Trainer of at least equal value to existing VR training models.16 Using the SimPORTAL model, Poniatowski et al.17 showed that average maximum forces for needle puncture into skin varied from 2.75 N to 2.80 N for human tissue, and from 4.53 N to 4.19 N for simulated human tissue.
There is evidence that hybrid ex vivo models of training with benchtop models used in conjunction with traditional senior-led teaching, direct supervision, and feedback on trainee performance can achieve greater competence and confidence in trainees in a shorter space of time than could ever be achieved with traditional in vivo training. A common drawback of these types of models is their inability to replicate the human factors involved with PCN (e.g., movement of the kidney with respiration and the true tactile experience of puncturing the flank and entering the perinephric space).
Augmented Reality and Virtual Reality
A prospective study performed by Papatsoris et al.18 looked at the impact of VR training of percutaneous renal access in a cohort of 36 urology trainees who had never performed the procedure before. The PERC MentorTM (3D Systems [formerly Simbionix USA Corp.,], Rock Hill, South Carolina, USA) was initially used by a consultant urologist to demonstrate renal access puncture and guidewire access (Figure 1A and 1B). This simulator mimicked percutaneous renal access with a 3D model of the patient’s flank, a virtual fluoroscopic C-arm, and pedals which simulated fluoroscopic screening. Ports allowed the introduction of guidewires and catheters into the simulator. The trainees were then given two 1-hour sessions, a week apart, on the simulator device, as well as a report of the statistics on their performance, such as procedural time, radiation exposure, volume of contrast used, and potential complications. This study found that the use of simulation was statistically significant in reducing the amount of radiation used by the trainees, as well as the time taken to perform the procedure. In addition, the time to gain desirable guidewire placement was halved after training. The PERC Mentor has been evaluated well, and face, content, construct, predictive validities, and skill acquisition by trainees have been reported.19-21 The cost of the simulator (100,000 USD) is the main reason for the lack of widespread acceptance.
The K181 VR surgical simulator (Marion Surgical, Niagara Falls, Ontario, Canada) allows users to interact with a virtual patient in a virtual operating room (Figure 2). The system has three main components: the VR headset places the user in a virtual operating room, and the haptic system offers the user with haptic force-feedback calculated by the tissue simulator. Twelve participants evaluated the simulator, and 95% reported realistic renal access simulation.22
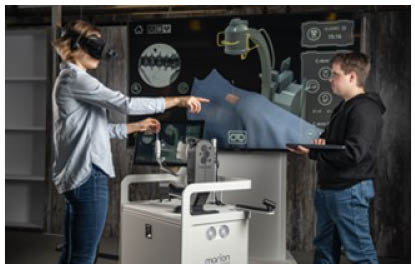
Figure 2: Marion Surgical K181 Simulator (with permission from Marion Surgical, Niagara Falls, Ontario, Canada).
Augmented reality (AR) and VR simulators offer additional features such as the ability to recreate a number of pre-programmed scenarios that may be encountered in practice but cannot be replicated with a benchtop model. These may help expose trainees to a range of potential complications, and allow for the practice of dealing with these when encountered in reality. The only other method of training that has shown evidence of helping trainees to deal with complications is live anaesthetised animal models, which are not often used in the UK because of ethical reasons. Levels of procedural difficulty can also be manipulated in AR and VR to allow for the development of not only basic technical skills, but also more advanced procedural skills, and confidence in a variety of scenarios. VR and AR allow for statistical analysis of performance, which can aid the training circle by providing ongoing feedback to the trainee, and evidence of progression in obtained skills.23
The Perk Tutor (Queen’s University, Kingston, Ontario, Canada) AR training system is used for teaching and assessing PCN using tracked-ultrasonography-snapshot (TUSS) technology. Four novice urology residents with no prior experience in PCN participated in a study as operators, and each operator completed two TUSS-navigated procedures and two conventional ultrasonography-guided procedures. TUSS-guided PCN was noted to be superior in several parameters, including the number of attempts, time taken, and amount of needle motion in tissue. The model is not commercially available due to a lack of validation.24
Live Anaesthetised Animal Studies
Studies that analysed live animal models have shown that most aspects of the real-life PCN procedure can be successfully replicated, adding a sense of realism to the training. Live porcine models have been rated as superior to simulators in terms of realism, movement of the kidney, and tactile feedback in studies.25 As with most biological methods of training, the main drawbacks are the limited use and inability to be used repetitively. In most UK institutions, use of live animals is difficult for ethical reasons.
Cadaveric Training
Cadaveric training, although a staple method of training in other aspects of surgical training, has few percutaneous access studies validating its effectiveness. It is a limited resource, and is not often widely available across different training sites. In addition, cadaveric methods of training do not provide haptic feedback, which is often essential in percutaneous intervention.
PROBLEMS FACED BY TRAINEES
Doctors in training who wish to learn nephrostomy tube insertion are certain to face a number of challenges. Increasing trainee numbers limits the number of learning opportunities during daytime working hours, and contemporary working patterns may be an obstacle in gaining hands-on experience outside of contracted hours. Some of the teaching methods (e.g., simulators), although generally well-received, come with their own limitations (Table 1). Their availability to trainees varies between hospitals and access can be limited. Furthermore, their actual usefulness remains to be proven. Simulators can certainly fill learning gaps in the initial stages of training, where trainees have no prior experience of the procedure at all, but for more senior trainees, their usefulness will vary. The realism they reproduce will always be secondary to that of the real-life clinical environment, and, therefore, ongoing validation studies are crucial to determine to what extent their use actually fulfills the learning requirements of trainees at different stages of training.26,27
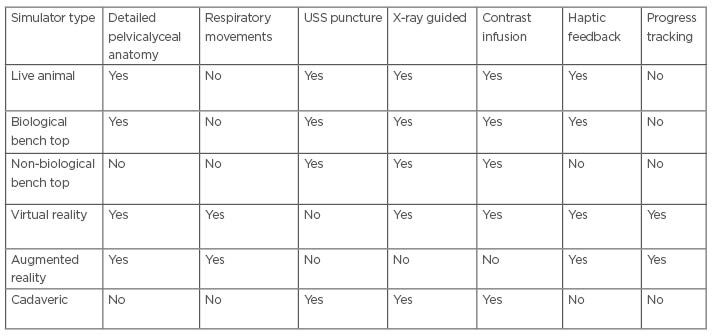
Table 1: Features of different simulation techniques for percutaneous nephrostomy.
USS: ultrasonography-snapshot.
In the authors’ institution, they conducted a survey amongst trainees to explore in greater detail the challenges they face in their training. The points that the exercise raised were in congruence with the above arguments. One respondent argued that being part-time often results in them missing days when intervention is happening. Variance in difficulty between cases, lack of prior training, having different supervisors each time, and issues with patient’s comfort during the procedure were also mentioned as challenges to training. Despite sessions taking place in a large teaching hospital, one trainee was concerned that the patient wasn’t counselled properly, and worried that teaching might make the patient uneasy during the procedure. Trainees also agreed that available simulation models are not as good as practicing in a real-life environment. One urology trainee pointed out that at present there is neither a requirement by the Joint Committee on Surgical Training (JCST) nor formal training for UK urology trainees to gain any exposure to or competency in PCN. Finding time alongside their usual commitments poses another challenge. Any exposure in theatre to PCN and PCNL access is often ad hoc and not formally recognised. This makes becoming proficient in ultrasound scanning and renal puncture challenging. Often, a radiology trainee will also be present at these lists, limiting the urology trainee’s exposure.
In an era where all specialties are feeling the pressure of increased workload with limited resources, it can be argued that training urologists in PCN and percutaneous renal access would be of benefit. This would have the benefits of freeing-up radiology colleagues from attending urological theatre lists, and giving greater freedom to schedule urological cases requiring PCN access without affecting radiology commitments. This is a common problem at the authors’ institution, where PCNL and antegrade procedures can only be performed on 1 day of the working week.
RECOMMENDATIONS
PCN training involves facing a steep learning curve, and researchers have argued that a resident has to perform approximately 24 procedures in order to be proficient.27,28 In their review of the literature on training in percutaneous nephrolithotomy, Mishra et al.20 described several categories where recommendations can be made in order to optimise training. They argued that before any formal training takes place, cognitive learning needs should be addressed, as most errors made by trainees in simulation usually result from their knowledge gaps rather than technical mistakes. They stressed the importance of a suitable, realistic, and risk-free training environment for the initial steps before practicing on patients, and recognised the usefulness of simulators for practice of repetitive tasking. They also argued that the most efficient wet-lab model is a live anaesthetised porcine, which closely replicates the human organ. The authors believe that all of these recommendations apply to trainees learning how to perform nephrostomies.
It is widely recognised that the most crucial task on which the procedure success heavily depends during nephrostomy tube insertion is the initial access. Training methods should emphasise this critical step. Establishing the correct depth of initial percutaneous needle insertion is widely recognised as the critical initial challenge. The 3-finger technique described by Shergill et al.29 is an example of a safe, cheap, and easy to learn method that can be used in teaching.
Some challenges faced by trainees will be unique to each institution, and therefore close supervision, feedback provision, and evaluation of the effectiveness of the adopted methods will undoubtedly be key elements. Conducting a simple survey amongst trainees can be a very useful tool. In the authors’ experience, trainees argued that sufficient training opportunities exist; however, they emphasised their preference to be taught directly on patients by consultants rather than on simulators during routine outpatient lists.
These results may in part be due to the limited access to other training modalities, such as benchtop models or simulators at the authors’ institution, and raise the question of whether the availability of such ex vivo training tools would have impacted the results of their survey.
The methods described within this overview allow for the training of a greater number of trainees in a safe, risk-free environment where repeated practice can be performed to not only achieve competence, but also confidence, in an era where training prospects are limited by the workforce pressures and limited training opportunities. Moreover, it highlights how medical education is ever-evolving, and traditional methods of training are being adapted with state-of-the-art technologies to provide a lifelike in vivo training experience without any clinical risk, albeit at a cost. The greater potential that this overview highlights is how methods of training used in surgery can also be utilised in other specialities to train in practical skills, with the potential for collaborative training and working (for example, between urologists and interventional radiologists). In the future, this may lead to a greater number of clinicians equipped with the skillset to perform such procedures independently, and has the potential to, therefore, alleviate stresses on acute services and make changes in the workforce.
CONCLUSION
Learning how to perform PCN can seem like a daunting task for doctors in training. It is undoubtedly an iterative process. Good understanding of the procedure, and repetition of the newly acquired skill with the aid of simulation and phantom models with direct feedback, followed by practice in a supervised clinical setting, are the fundaments of an effective learning process. The authors believe that there is a role for ex vivo training tools, which can not only increase early exposure to the steps of PCN in a safe, risk-free environment, but can be used to train greater numbers of trainees than in vivo cases can. This method of training may, therefore, help to increase the number of radiology and urology trainees trained in PCN by levelling out opportunities to perform PCN in a classroom setting, with the overall potential to help streamline the intraoperative process for endourological procedures, as well potentially alleviating the burden of the interventional radiologists on call. Formal guidelines for establishing proper training sites do not exist, and therefore success will frequently depend on goodwill of mentors.