Abstract
Sperm cryopreservation has been widely used for assisted reproductive technology (ART). Indications for sperm cryopreservation include donor insemination, cryopreservation prior to surgical infertility treatment, and malignancies to avoid additional surgery in couples undergoing repeated in vitro fertilisation (IVF) or intracytoplasmic sperm injection (ICSI) cycles. However, dramatic changes during cryopreservation have detrimental effects on the sperm membrane, resulting in a large increase in the percentage of poorly motile sperm or sperm with abnormal morphology. The negative effects related to rapid temperature decrease, such as osmotic injury, cellular dehydration, intracellular ice crystal formation, and oxidative stress can also damage the sperm in ways that affect reproductive outcome.
This comprehensive review focusses on describing the detrimental effects of the cryopreservation process on sperm and aims to clarify that not all impaired sperm parameters have the same impact on the clinical practice of ART. Regarding the parameters studied, some of the biomarkers used for sperm maturity, hyaluronic acid binding capacity, or damaged DNA have limited clinical significance compared to other semen parameters which provide more useful information for clinical practice and are often dismissed, such as total motility or total motile sperm count (TMSC). In the authors’ experience, TMSC gives valuable quantitative information about the number of viable spermatozoa. Indeed, TMSC should be assessed specifically for groups of patients in which sample availability is limited.
INTRODUCTION
The cryopreservation of gametes, as well as more complex multicellular organisms such as embryos, is an increasingly common practice in the field of assisted reproduction technology (ART) and reproductive medicine. Cryopreservation is described as the process of long-term cell preservation and storage at cryogenic temperatures (-196 °C), thus maintaining cell viability by means of hampering ageing and cell degradation processes.
Recent advances in the field of cryobiology have not only led to the development of novel fertility treatments, such as fertility preservation and gamete/embryo donation, but also helped co-ordinate gamete retrieval and fertilisation measures.
Since its early beginnings in the 1970s, human sperm cryopreservation has been demonstrated to be a crucial aid in ART. Currently, many male patients attend sperm cryopreservation programmes for fertility preservation purposes.
The cryopreservation and storage of human ejaculated spermatozoa is commonly facilitated in groups of male patients reporting difficulties in semen sample collection, such as in cases of erectile dysfunction and lack of or retrograde ejaculation. Additionally, to schedule oocyte retrieval and semen sample collection, sperm cryopreservation is counselled in those male patients who will not be able to collect the sample the day of ovarian puncture.
Furthermore, sperm cryopreservation should also be considered in cases of azoospermia to prevent repeated sperm retrieval surgeries. Similarly, cryopreservation is also recommended for groups of male patients who have impaired semen parameters, or in cases in which there is an observed, progressive decrease in sperm quality, to prevent the risk of azoospermia.1
Additional to these purposes, male fertility preservation is particularly relevant in oncologic patients, or in patients reporting chronic non-malignant diseases or autoimmune disorders. The gonadotoxic effect of treatments is the main reason why fertility preservation is counselled in such patients, since chemo/radiotherapy regimens as well as other medications for chronic diseases are a real threat to fertility potential.2,3 Indeed, in some cases sperm parameters are impaired even prior to any treatment. This could be in response to the direct impact of the tumour on the male genital tract, as is observed in testicular cancers. Additionally, indirect effects inherent to oncological processes could also be a plausible cause of sperm parameter impairment in these patients.4
As the survival rates of patients living with malignant or non-malignant chronic disorders are increasing as a result of the new therapeutic options available to them,5 there is increasing interest from young male patients of reproductive age for fertility preservation. Since long-term quality of life following cancer treatment has become more prevalent in society, initiating semen cryopreservation before gonadotoxic treatments is seen as an effective means of maintaining male reproductive potential. A study carried out by Duadin et al.6 investigated the growing trend to cryopreserve the sperm of pubertal boys and young adults being treated for leukaemia, lymphomas, or other types of germ cell tumours.
Moreover, it is worth mentioning that the implementation of sperm cryopreservation protocols enables the establishment of donor sperm banks and, consequently, donor sperm programmes.
Unfortunately, despite the many strengths of sperm cryopreservation, important damages to the sperm cells are still reported. Furthermore, compared to other methods there is currently no standardised cryopreservation protocol for human spermatozoa that leads to higher viability yields after thawing.
The aim of this review is to provide comprehensive information regarding the state-of-the-art human spermatozoa cryopreservation techniques, highlighting deleterious effects associated with freezing and vitrification protocols.
METHODS
An extensive literature review on the effects of sperm cryopreservation on sperm cells was performed. Topic-relevant scientific papers published in PubMed and Google Scholar databases were included in the current comprehensive review. Key words used for the bibliographic search were “sperm freezing”, “male cryopreservation”, “vitrification”, “cryodamage”, and “cryosurvival”.
Cryopreservation of Human Spermatozoa
The cryopreservation of sperm cells is not a harmless process; indeed, the significant damage of spermatozoa due to freezing and vitrification protocols is well-described. Cryoinjury, which is the damage associated with cryopreservation, leads to an impairment of sperm parameters. In addition, according to Honda et al.,7 the ageing process is not totally prevented by cryopreservation. They suggest that during cryopreservation the telomere-shortening phenomenon is observed, as well as an induction of cellular senescence.
Changes in Sperm Cell During Cryopreservation
The sperm cell membrane is a vital structure during the freezing and vitrification processes since cell survival is strongly dependent on its viability, especially during the temperature decrease and following the return to physiological temperature by thawing. The sperm cell membrane exhibits as a fluid mosaic-like structure, presenting a phospholipid bilayer with protein and carbohydrates dispersed throughout. The respective stiffness or fluidity of the sperm cell membrane is determined by the fatty acids present in the phospholipid bilayer.
The membranes of human spermatozoa are characterised by their large number of polyunsaturated fatty acids (PUFA), which are responsible for membrane fluidity. Unsaturated lipids, together with the presence of cholesterol, impair the phospholipid packaging, thereby decreasing the number of possible interactions between phospholipids. As a result, membrane fluidity is increased due to steric effects. Increased sperm cell membrane fluidity ensures a better response to the cryopreservation process,8,9 and therefore a large number of PUFA translates into a greater protection against cryoinjury during cryopreservation.10 As well as fluidity, permeability is another key feature of the sperm cell membrane. Permeability of the sperm cell membrane will continually decrease during the freezing stage of cryopreservation to the point where the membrane becomes an impermeable structure; hence, all fluids retained in cell cytoplasm will become vulnerable to crystalisation.9
A crucial parameter during cryopreservation is the freezing rate. During the temperature decrease from physiologic temperature (37 °C) to 0 °C, a temperature shock occurs. This initial temperature decrease promotes the change of phase of membrane phospholipids from the fluid to gel phases. As the phase exchange is not simultaneous across the whole phospholipid bilayer, both phases can be observed before completion. The coexistence of both phases, fluid and gel, leads to an impaired phospholipid arrangement, increasing the cell’s permeability to solutes. The use of cryoprotectant agents and a progressive freezing rate minimises this damage during the first freezing step.
The following temperature decrease below 0 °C promotes intracellular ice crystal formation. It is therefore paramount to induce a major degree of cell dehydration, so as to minimise the water content inside the sperm cell, because ice crystals cause physical damage to organelles and cell membranes. A specific, optimum freezing rate is associated to each cell type in which cryoinjury is minimised. Supra-optimal freezing rates promote ice crystal formation as a result of incomplete cell cytoplasm dehydration, whereas osmotic damage due to the high solute concentration in the extracellular medium is associated with sub-optimal freezing rates.11
In parallel to the temperature decrease, a calcium influx towards the sperm cell cytoplasm is observed. Under this circumstance, phospholipids present in cryoprotectant agents contribute to membrane resistance to calcium shock,12 and therefore an appropriate balance in calcium levels is important to ensuring good post-thawing recovery. As well as the temperature decrease, high calcium levels in the extracellular media help promote cellular damage during the cryopreservation procedure.13
Assessment of the Cryopreservation Process
Cryopreservation-associated damage to the spermatozoa not only alters the cell membrane structure,9,14,15 but also the cell’s metabolism and mitochondrial bioenergetic processes.16,17 After thawing, protein degradation and phosphorylation have been reported.18 Interestingly, capacitation-like changes to spermatozoa are associated with protein phosphorylation.18 It is noteworthy that the lifespan of spermatozoa exhibiting capacitation-like features is shortened and, in combination with the decrease in spermatozoa with intact acrosomes after thawing, this fact would reinforce the hypothesis that the fertility potential of frozen-thawed spermatozoa is threatened.
All these cited changes have an impact on sperm parameters, such as decreasing motility or altered morphology. Furthermore, as well as mitochondrial damage, impaired motility, and morphology, sperm DNA integrity can be affected during cryopreservation.19 As Figure 1 shows, the cited parameters are the main targets of cryodamage.
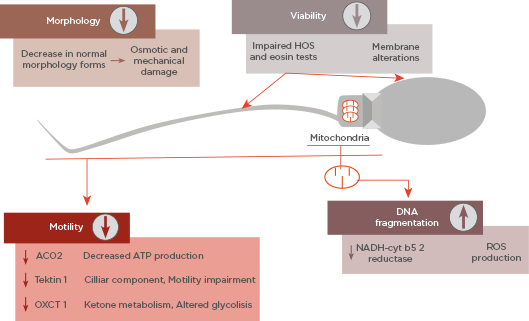
Figure 1: Summary of main sperm parameters affected by cryodamage and its causes.
ATP: adenosine triphosphate; HOS: hypo-osmotic swelling; ROS: reactive oxygen species.
Sperm Morphology
The effect of cryopreservation on sperm morphology has been the subject of a wide range of studies. Some studies demonstrate a decrease in normal morphological forms in the semen sample after thawing.14,20-22 When slow freezing is considered, it is suggested that the osmotic shock caused by cryoprotectant agents required in this protocol would be the main reason for observing altered morphological forms in the thawed samples, whereas when vitrification is the chosen cryopreservation protocol, the impairment of normal morphology would be associated to extracellular ice crystal formation.22 However, altered sperm morphology has no clear impact on reproductive outcomes, as recently reported in a number of studies.23-25 It should also be noted that, when it comes to the assessment of sperm morphology, technical limitations lead to the hinderance of result interpretation due to intra and inter-laboratory differences.
Sperm DNA Integrity
Cryopreservation-associated alterations to sperm DNA are mainly the result of two processes: abortive apoptosis and oxidative stress caused by reactive oxygen species (ROS). According to Thomson et al.,26 ROS production could be the main cause of damage to sperm DNA during cryopreservation.
With regard to ROS production, endogenous ROS release is common in human spermatozoa because superoxide anion dismutation leads to hydrogen peroxide production. The cytotoxic effects of hydrogen peroxide are well-established, as seen by its high oxidant activity. In this sense, as has been suggested previously by Meseguer et al.,27 high levels of glutathione-peroxidases could be a biomarker for success after cryopreservation procedures since their activity consists of eliminating DNA damaging free radicals.
In parallel, other components found in seminal plasma, such as antioxidant enzymes, prevent ROS release. Similarly, the protective role of albumin against endogenous ROS production has been reported.19,26 Albumin’s protective role involves its capability for binding lipid peroxides and neutralising their cytotoxic effects.28
However, the clinical impact of cryopreservation-associated DNA fragmentation is still a debatable issue, as suggested in recent reviews and meta-analyses.29,30 Differences among samples regarding cryoresistance, protocols for cryopreservation, and the wide range of methods used to assess DNA integrity make the agreement of a consensus difficult.
The meta-analysis carried out by Robinson et al.31 puts forward the idea of an increased risk of miscarriage being associated with the use of high-fragmented spermatozoa.31 Unfortunately, as the authors also commented, there are design flaws in the studies included in the meta-analysis. Firstly, the threshold values for DNA fragmentation index varies among the studies, from 10–36%.32,33 Even using a high sperm fragmentation index, Esbert et al.33 did not observe any differences in clinical outcomes, regardless of the IVF technique used and the origin of the oocytes. In addition, the results clearly show a dependence of risk ratio’s ranges on the test used for DNA fragmentation assessment, and thus important variations among the risk ratios are observed when different DNA fragmentation assays are used. Another common limitation regarding the assessment of sperm DNA fragmentation and clinical outcomes is the cohort of oocytes included. An elegant study carried out by Meseguer et al.34 defends the premise that oocyte quality is a major factor to consider due to the ability of good-quality oocytes to conditionally repair DNA damage. Therefore, this should be considered when it is stated that sperm DNA damage worsens reproductive outcomes through an increase in miscarriage rate in clinical studies including poor quality oocytes.35
Addressing the issue of using frozen or fresh spermatozoa for ART purposes, Ribas-Maynou et al.36 previously reported a slight increase in single-stranded DNA lesions when cryopreserved spermatozoa were used, but no difference could be established, in terms of double strand DNA damage, between fresh and frozen sperm samples. This is particularly important because different sorts of DNA damage have different clinical outcomes.37,38 Consequently, double-stranded DNA breakages are associated with miscarriages, while single-stranded DNA lesions have no further clinical impact except a putative decrease in fertilisation rate and a delay on pregnancy achievement.39 Moreover, comparative studies of different cryopreservation protocols fail to find differences in sperm DNA integrity among the protocols studied.20,22,40-44 More remarkable is that most of these studies reported a high degree of DNA integrity, superior to 70%, after cryopreservation.20,40,41,43,44 In contrast, some of these studies observed lower sperm DNA integrity, in the range of 15%, in thawed samples.22,42 However, it is relevant to note that the sperm DNA integrity prior to cryopreservation is not available in these cases.
Sperm Maturity
To date, several maturity markers have been reported for sperm cells. In brief, high cytoplasmic levels of creatine kinase (CK) correlate with the spermatozoon’s immaturity, suggesting an impairment in the last phase of spermatogenesis, when the cytoplasm extrusion occurs. Moreover, high expression of chaperonin HspA2, in combination with low levels of CK, are biomarkers of sperm maturity.45
At least three hyaluronic acid binding proteins have been described in mature spermatozoa. These proteins are membrane receptors that are involved in relevant physiological processes, such as acrosome reaction, hyaluronidase release, and the binding to the oocyte’s zona pellucida.46,47 Therefore, a sperm maturity profile has been developed based on the capability of spermatozoa to bind hyaluronic acid. In the research carried out by Yogev et al.,48 spermatozoa maturity status has been used as a marker of successful cryopreservation outcome.48 These authors report a poor correlation between spermatozoa hyaluronic acid-binding capacity and survival rates in thawed samples. According to these evidences, hyaluronic acid binding sites in spermatozoa are not altered by cryopreservation; hence, hyaluronic acid-binding capability would not be a useful marker to assess cryopreservation outcomes.47,49
Sperm Survival: Viability and Motility
Sperm survival is the main parameter to consider when assessing cryopreservation outcomes. Traditionally, sperm survival is assessed by different assays with the same biological basis: sperm cell membrane integrity.
Standardised viability tests are based on the assessment of sperm cell membrane’s integrity as spermatozoa exhibiting altered cell membranes are associated with the loss of cell functionality. Eosin staining and the hypo-osmotic swelling test (HOS-test) allow to distinguish viable, but not motile, spermatozoa; hence the use of the cited tests is interesting in clinical cases in which sperm motility is difficult to assess. Several studies agree that, regarding the number of viable spermatozoa after thawing, it is because of physical and chemical stress that cryopreservation procedures worsen the results derived from those viability tests.15,20,50,51
Despite strengths, these classic viability tests are not exempt from limitations. Eosin staining does not allow the use of the stained sperm sample in the following artificial reproduction techniques; hence the putative information derived from eosin staining results cannot be fully translated to the clinical practice when it comes to selecting the spermatozoon to be microinjected. Alternatively, after HOS-testing, the spermatozoa remain viable because the biological basis of HOS-test relies on the sperm cell’s swelling capacity as a response to preserve osmotic balance under hypo-osmotic conditions. As hypo-osmotic conditions are established in vitro by exposing spermatozoa to a relatively harmless solution of sodium citrate and fructose, thus maintaining sperm viability, the sample could be used in the following ICSI, allowing the selection of viable spermatozoa exhibiting swelled tails.
However, some studies suggest that the spontaneously developed tail swellings occur in some cases under physiological conditions, resulting in an increased false positive rate because the tail swelling occurs in the absence of hypo-osmotic solution. Hossain et al.50 also reported that the spontaneously developed tail swelling phenomenon is particularly increased in thawed samples.
On the other hand, biologically, the movement of sperm cells is fuelled by means of energy from adenosine triphosphate (ATP). One of the sources of ATP can be the oxidative phosphorylation carried out by mitochondria located in the mid-piece of human spermatozoa. In spite of some concerns about the putative transference of ATP derived from mitochondrial activity through the flagellum, it could be hypothesised that ATP released from oxidative phosphorylation by mitochondria could be transported by means of the flux transfer chains, a model published by Dzeja and Terzic.52 Other authors support this hypothesis,53 and the decrease in sperm motility in certain circumstances in which mitochondrial function is inhibited is additionally represented in robust evidences.54-57 Nevertheless, ATP used for the movement of spermatozoa is not exclusively produced by oxidative phosphorylation in the mitochondria. Indeed, glycolysis is another source of ATP that fuels sperm motility.58-60 In fact, a recent comprehensive review addressing the issue of the signalling pathways involved in sperm motility suggest that, possibly, both mentioned mechanisms co-operate to allow ATP production for sperm motility.61 The degree of contribution of each metabolic pathway could depend on the substrate’s availability in the female reproductive tract or in the culture media.
Several authors agreed that a decreased motility rate can be observed, as well as decreases in other kinetic parameters, in thawed samples.14,16,17,21,22,62-64 The observed decrease in sperm motility could be due to the mitochondrial damage described after cryopreservation, as less functional mitochondria with reduced activity have been reported in thawed samples.16
In this sense, sperm motility could provide a relatively accurate estimation of viability provided the premise that a motile spermatozoon is viable. The Computer Assisted Semen Analyzer (CASA) system can assess more accurately than manual observation the motility of a given sample, because this sort of software considers all types of motility that a spermatozoon can exhibit and is not only limited to progressive motility traditionally assessed by manual observations. Indeed, sperm total motility (TM) strongly correlates with HOS-test results in terms of sperm viability.65 Therefore, TM could be used as an estimation of the viability of a given sperm sample. TM assessed by CASA systems could be considered a rapid and objective method to estimate cryopreservation outcomes regarding sperm survival. However, there exist some exceptional clinical circumstances in which sperm viability tests, such as the HOS-test, cannot be replaced. In patients reporting an absolute structural alteration of the sperm flagellum, as in Kartagener syndrome, the HOS-test should be used rather than other assessments of sperm motility due to the physical incapability of the spermatozoa to move.66,67
Total Motile Sperm Count
Sperm count is also a paramount parameter that should be considered in certain clinical scenarios, particularly when the sample’s availability is limited. The combination of TM percentage and sperm count in a single parameter provides useful information, not only about viability rate, but also quantitative valuable information regarding the total number of viable spermatozoa.
A quantitative assessment of the number of viable spermatozoa is especially crucial in groups of sub-fertile patients reporting impaired sperm count or motility, as in these circumstances it is vital to maximise the number of motile spermatozoa achieved. Recent studies agree that using total motile sperm count (TMSC) as a sperm parameter contributes to achieving better outcomes in different clinical cases, such as in testicular cancer,68 severe male factor diagnosis,69 and in ICSI cycles.70
Differences in the clinical impact associated with each sperm parameter included, based on the main evidences included in this review, are summarised in Figure 2.
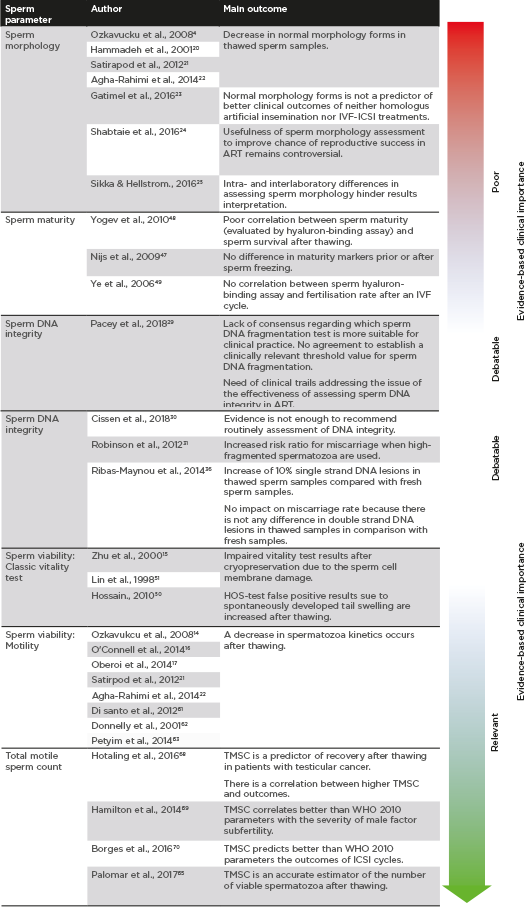
Figure 2: Clinical importance of sperm parameters based on published evidences.
ART: assisted reproductive technology; ICSI: intracytoplasmic sperm injection; IVF: in vitro fertilisation; TMSC: total motile sperm count; WHO: World Health Organization.
CONCLUSIONS
Cryopreservation of human spermatozoa is a process that causes severe damage to sperm cells. Even though there are several sperm parameters that can be the target of cryodamage, the clinical impact of each parameter is not the same. While the clinical usefulness of some parameters on reproductive outcomes is unclear or remains controversial, such as sperm morphology, sperm maturity, or DNA integrity, other sperm parameters, including TMSC, should not be dismissed, and should be strongly recommended to be assessed in certain clinical circumstances when the sample’s availability is a limiting factor.