Abstract
Noninvasive ventilation (NIV) is frequently used in patients with acute respiratory failure and its success is dependent on the underlying cause of the condition. When used for cases with a more rapid, reversible nature, like cardiogenic pulmonary oedema or acute exacerbations of chronic obstructive pulmonary disease, early intervention before patient deterioration is a key factor in success. Gastric distention-associated anastomose leakage after bariatric surgery is overestimated and the success of NIV trials in patients with encephalopathy has a strong association with the triggering cause rather than the severity of a coma. Immunocompromised patients mostly benefit from a short period of ventilation and more invasive ventilation is associated with excessively high mortality independent of the cause. In other diseases with parenchyma inflammation or infection, little success with NIV has been shown. Limiting ventilator-induced lung injury in these patients is another issue and is mostly achieved with heavy sedation or paralysis. Since NIV failure increases the risk of mortality, determination of a failing patient is of paramount importance. Clinical and laboratory surrogates of muscle fatigue can also be assessed. Adequate pressure support and positive end-expiratory pressure levels vary and the haemodynamic status of the patients must be considered. Ventilator–patient asynchrony increases NIV failure. Unfit interfaces also result in asynchrony, which will inevitably lead to failure, and observing waveforms can address this issue. The aims of this review were to understand the mechanism of NIV that leads to its failure or success, to become aware that delaying the appropriate therapy increases mortality, and to elucidate that spontaneous breathing can be a double-edged sword in some circumstances.
INTRODUCTION
Noninvasive ventilation (NIV) has been used for many years and its use has been steadily rising in the last two decades. Apart from substantial evidence supporting its use in specific clinical problems, like cardiogenic pulmonary oedema or acute exacerbations of chronic obstructive pulmonary disease (COPD), there are many controversies regarding the use of NIV as a first-line ventilatory support therapy in other types of acute respiratory failure (ARF), such as community-acquired pneumonia or acute respiratory distress syndrome (ARDS). As NIV failure may increase mortality, it is essential to have a good understanding of the respiratory pathophysiology before generalising the success of the procedure to other situations.
When the cause of the ARF is reversible and there is a need for ventilator support (i.e., the patient shows signs of muscle fatigue), NIV is often initiated. It can be used to avoid intubation and its complications, to reduce work of breathing (WOB), to prevent atelectasis, to assist the failing heart, and to enable time for the treatment of the underlying disease.1 However, reverting from spontaneous negative-pressure ventilation to positive-pressure ventilation (PPV) has its consequences, and the underlying pathology of the ARF is a significant factor in the success of NIV.
POSITIVE-PRESSURE VENTILATION AND HEART–LUNG INTERACTIONS
Traditionally, the lung can be divided into three Zones of West; although this is an oversimplification and there are many more factors influencing ventilation and perfusion in the lungs, it is helpful to understand and predict changes with interventions.2 In Zone 1, there is ventilation but no perfusion, which is termed dead space ventilation. In a healthy lung, there is no Zone 1 and most of the lung consists of Zone 3, so perfusion in the lungs is independent of the pressure within the alveoli. PPV increases alveolar pressure and may enlarge Zone 1, thus leading to dead space ventilation. NIV, especially with full face masks, also increases apparatus-associated dead space.3 These two factors may lead to CO2 accumulation, which may not be overcome by increased minute ventilation. In situations when the respiratory pattern becomes rapid and shallow, this also must be considered.
PPV also induces right ventricular (RV) dilatation and increases its afterload, thus increasing the work performed by the RV. In cases where RV workload has risen, like pulmonary thromboembolism, pulmonary hypertension, or an RV infarct, one must be careful about the deleterious effects of PPV. Hypoxaemic pulmonary vasoconstriction also increases pulmonary vascular resistance (PVR) and RV workload, so a precise balance between these two endpoints must be considered.4
PVR has two main components, alveolar vessels and extra-alveolar vessels, and is lowest at the functional residual capacity. Below this volume, towards residual capacity, hypoxaemic pulmonary vasoconstriction increases together with PVR and, with increasing lung volume, the alveolar pressure increases and compresses the alveolar vessels, again increasing PVR.
Increases in intrathoracic pressure are reflected as an increase in right atrial pressure, and this impedes venous return to the heart. Decreased venous return to the right ventricle decreases left ventricular preload, which may reduce the cardiac output of the left ventricle. Increased PVR and RV dilatation push the interventricular septum against the left ventricle, impairing its preload further. Therefore, in haemodynamically unstable patients, instituting PPV may lead to circulatory collapse. Adequate hydration can limit these effects, but a strict negative fluid balance may be harmful to these patients because West Zone 3 becomes West Zone 2, which makes alveolar perfusion dependent on alveolar pressure, increasing the ventilation–perfusion mismatch further.5
CHRONIC OBSTRUCTIVE PULMONARY DISEASE
Acute exacerbations of COPD patients have an increased WOB, muscle fatigue, and respiratory acidosis. The pressure support delivered with NIV helps to unload respiratory muscles, and the external positive end-expiratory pressure (PEEP) applied to the lung assists the patient to overcome intrinsic PEEP (iPEEP). Mechanisms leading to iPEEP include an increased time constant for expiration due to expiratory flow limitation in COPD patients and a short expiratory time resulting from the higher respiratory rate. Inability to exhale the volume in the alveoli leads to dynamic hyperinflation, which disrupts respiratory mechanics. When the patient initiates a breath, first the excess alveolar pressure must be overcome, then a negative pressure must be generated to create a gradient between the airway, referred to as zero pressure in a spontaneously breathing subject, and the alveoli, allowing gas flow to the lungs. This extra load leads to respiratory muscle fatigue and is a significant contributor to increased WOB in COPD patients. External PEEP application may reduce the gradient between intra-alveolar pressure and the airway opening pressure. In a patient with an iPEEP of 10 cm H2O and a PEEP of 5 cm H2O, the patient must first level their excess pressure with the airway opening pressure (assumed to be 5 cm H2O) when the ventilator is still in the expiratory phase and is thus unable to assist. If external PEEP is increased to 8 cm H2O, the new gradient between the intra-alveolar pressure and airway opening pressure becomes smaller. In this way, applying external PEEP to assist patients in initiating a breath is a common method. iPEEP is measured in a no-flow state, namely with expiratory hold, and is hard to perform in a spontaneously breathing patient. Thus, observing the expiratory flow waveform and the point where the patient initiates a breath, and its distance from the zero line, will help identify internal PEEP and its response to external PEEP. Other than this, changing pressure support levels and/or mandatory ventilation frequency or the I:E ratio will also address this issue. It is important to keep in mind that applying external PEEP to assist the patient in triggering the ventilator does not modify the dynamic hyperinflation unless a greater external PEEP value than iPEEP is set. If a higher external PEEP is used, this will increase dynamic hyperinflation and can exacerbate the working conditions of the respiratory muscles.6 It is evident that a ‘one size fits all’ level of 5 cm H2O PEEP will have a different effect on every patient and must be titrated. Analysing flow waveforms on the ventilators would address this issue.
In a large retrospective study, NIV was associated with better outcomes compared to invasive mechanical ventilation (IMV) in acute exacerbations of COPD.7 On the contrary, another study of 9,716 COPD patients in the UK showed higher mortality in NIV patients.8 The investigators emphasised the importance of careful patient selection with milder respiratory acidosis and early start of therapy; severe acidosis (i.e., pH <7.26) and delayed treatment initiation led to NIV failure. Other risk factors associated with NIV failure were weak cough reflex and excessive secretions, hypercapnic coma, and patient–ventilator asynchrony.9
COPD without acidosis should not be treated with NIV to prevent the development of acidosis, and the mainstay of the therapy should be medical.10 A group of patients with COPD presenting with a recent onset of shortness of breath with mild acidosis (i.e., pH >7.30) were randomised to a standard therapy of inhaled beta-agonists, inhaled ipratropium, and/or steroids, or to NIV therapy; there was no reduction in worsening respiratory distress rate, though there were crossovers in the study. The authors also showed an almost 50% intolerance to NIV sessions. This study suggests that without overt respiratory distress or severe respiratory acidosis, initiation of NIV has no advantage over conventional medical therapy.11
ENCEPHALOPATHY
Due to a lack of airway protective reflexes and co-operation, severe hypercapnic encephalopathy is considered a contradiction to NIV. Milder hypercapnic encephalopathy in patients with COPD exacerbations (Glasgow coma scale [GCS] >10) can rapidly improve with NIV; however, a lower GCS, an APACHE II score ≥29, a respiratory rate ≥30, and a pH <7.25 on admission have been shown to have a risk of failure >70%.12 Pressure control ventilation or a high back-up rate can be used to reach the desired alveolar ventilation. Also, more CO2 binds to haemoglobin at lower oxygen saturation, increasing the CO2-carrying ability of the venous blood. This is known as the Haldane effect and means that blood oxygen saturation levels of 88–92% should be targeted.13
In other types of encephalopathies with hypercarbia, such as neuromuscular diseases associated with respiratory failure, the following must be considered before initiating NIV therapy: ability to clear secretions, the absence of agitation or agitations managed with conscious sedation, and expertise in NIV.14 A study conducted by Díaz et al.15 showed even higher success in patients with GCS ≤8 compared to GCS >8. However, the groups were not homogenous, and the more comatose patients had more NIV-responsive diseases like COPD (69.5% versus 25.5%). Overall, the outcome of NIV therapy in encephalopathy is dependent on the underlying cause rather than the level of encephalopathy.
CARDIOGENIC PULMONARY OEDEMA
Acute respiratory failure in cardiogenic pulmonary oedema (CPO) is due to muscle fatigue induced by an increase in WOB resulting from sudden flooding of the alveoli, an increase in airway resistance, a drop in the lung volume, and a decrease in oxygen diffusion capacity. Application of PEEP recruits alveoli, decreases venous return, and decreases left ventricular afterload, improving stroke volume. These are translated into a favourable effect and NIV is therefore recommended. In CPO, NIV is associated with a reduced need for intubation and decreased mortality.16 In addition, NIV in patients with acute coronary syndrome and ARF is not associated with an increased risk of cardiopulmonary events when compared with medical care.17
The ventilation mode, whether continuous positive airway pressure (CPAP) or bilevel positive airway pressure, is dependent on the underlying cause. Hypercapnic patients can benefit from increased alveolar ventilation and CPAP is effective in hypoxaemic patients, though a typical clinical presentation is uncommon. The superiority of one mode over the other has not been shown and the choice should be made separately for every patient.17
IMMUNOCOMPROMISED PATIENTS
A variety of conditions can affect immunocompromised patients and lead to ARF, such as opportunistic infections, drug-induced toxicity, and malignancy-related pulmonary damage. Immunocompromised patients are considered high-risk and extremely high mortality is associated with IMV; therefore, irrespective of the cause of the ARF, these patients may benefit from a trial of NIV to avoid complications related with IMV.18 However, a study including 115 ARF patients showed that a high-flow nasal cannula was associated with better outcomes compared to NIV. The authors associated this result with better patient tolerance and an absence of ventilator- induced lung injury (VILI).19
HYPOXAEMIC RESPIRATORY FAILURE
The response to NIV in hypoxaemic ARF patients can change with the underlying cause and comorbidities. In pneumonia patients without previous history of cardiac or pulmonary diseases, NIV was associated with a faster improvement compared to oxygen therapy in the arterial oxygen partial pressure (PaO2):fractional inspired oxygen (FiO2) ratio and a reduced intubation rate.20 However, the higher mortality rate in the rescue noninvasive group showed that delaying therapy worsens outcome. Another study also found improvement in oxygenation with NIV in community-acquired pneumonia patients compared to standard oxygen therapy, but oxygenation levels declined as soon as the NIV was stopped.21 It is possible that applying CPAP to lungs recruited collapsed alveoli caused by the inflammatory process and exudative flooding; however, once the positive pressure was removed, this effect was lost. The authors suggested that CPAP should be applied over longer intervals to allow time for antibiotics to have an effect.22
In patients with comorbidities, success rates were higher than the former group.22 On the contrary, no reduction in mortality or length of hospital stay compared to venturi mask was found in another study in patients with community-acquired pneumonia.23 Concern in these patients comes from higher mortality associated with NIV failure compared with immediate IMV.22 Patients should be selected with utmost caution and immediate IMV should be performed if the NIV fails to achieve the target (i.e., improved oxygenation).
Patients with ARDS also have a high rate of NIV failure, and the pressure levels needed to treat ARDS can be an indication for IMV. In an early study conducted in hypoxaemic patients, CPAP slightly improved oxygenation compared to oxygen therapy alone.24 The authors also suggested that the assessment of oxygenation at 1 hour of therapy (i.e., PaO2:FiO2 <200) can be used as a marker of severity.24
If NIV is selected for ventilatory support in hypoxaemic ARF patients, appropriate PEEP levels must be applied. There is great debate regarding what the appropriate PEEP level is, and the clinician must choose one of the several conflicting results from the studies conducted on this topic.25,26 Higher PEEP levels increase leaks and patient discomfort. It is also suggested that NIV should be reserved for patients with milder hypoxaemia (i.e., PaO2:FiO2 >200).27 NIV in this setting was associated with higher tidal volumes compared to IMV. Also, lower PEEP levels were applied in the NIV group, which can be adjusted to a certain limit, compared to invasive ventilation. This leaves increasing FiO2 as the only option to improve oxygenation. The result was higher mortality in NIV failure patients compared to severe ARDS patients managed with invasive ventilation.27 Also, a high tidal volume after 1 hour of NIV therapy was independently associated with mortality at Day 90.28
Higher PEEP application in NIV is linked with poor patient tolerance and increased leaks through the interface. The helmet interface has been used to address the problems of face mask application in hypoxaemic patients. In a study by Patel et al.,29 the helmet interface was shown to significantly reduce intubation rates (by >40%) and mortality compared to a face mask in hypoxaemic respiratory failure patients. The authors attributed this effect to continuous and better application of higher PEEP rates, though the difference between the groups was only modest (8 cm versus 5 cm H2O).29
NONINVASIVE VENTILATION AND LUNG INJURY
When the underlying pathology necessitating support is an injured lung, some receptors, such as the juxtacapillary receptors or central and peripheral chemoreceptors, may stimulate the respiratory drive.30 Whereas a healthy lung can tolerate considerable increases in tidal volume, as seen in exercise, lungs with pre-existing injury are susceptible to VILI.31 Ventilation-induced lung injury can be divided into two categories: VILI and patient self-inflicted lung injury.31 The concept of ‘baby lung’ is well established and measures have been taken to reduce the overdistention of the already-aerated alveoli. Protective ventilation with lower tidal volumes according to body weight can limit this overdistention, though it is an indication of a patient’s healthy lung size. Limiting driving pressure (the ratio of tidal volume to respiratory system compliance) also reduced mortality in ARDS patients.32 The transpulmonary pressure (TPP) is the alveolar pressure minus the intrapleural pressure and increases in TPP can lead to VILI.33 The TPP swings are similar for a given tidal volume, whether controlled, spontaneous, or partially assisted.31 In an inhomogeneous lung, the TPP swings can be regionally different34 and they are not always well reflected by a global measurement of tidal volume or TPP. A strong patient effort in patients with a higher respiratory drive may lead to the pendelluft phenomenon, which over-distends the more compliant alveoli.35 Limitation of tidal volumes may reduce lung injury, but this is hard to accomplish in severe patients. Moreover, in controlled ventilation, alveolar pressure is higher than the end-expiratory pressure during most of the respiratory cycle. This is not the case in spontaneous breathing, during which the alveolar pressure can drop below the end-expiratory pressure. With the increased vascular permeability seen in patients with injurious lung, thus having a higher respiratory drive, it increases the risk of pulmonary oedema.36 Therefore, these findings show that spontaneous breathing with higher tidal volumes can amplify lung injury, hence this is termed patient self-inflicted lung injury.31
Assisted spontaneous breathing has many advantages compared to controlled ventilation, including preserved diaphragmatic activity,37 better ventilation–perfusion distribution,38 better haemodynamics,39 and a reduced need for sedatives.31 On the other hand, the potential of increased respiratory drive to harm the lungs has been described in experimental conditions,40 and research has shown a reduced mortality in controlled ventilation patients.41 Decreased ventilator dys-synchrony, decreased respiratory drive, reduced atelectotrauma, and direct anti-inflammatory effects of cisatracurium were proposed to explain these findings. Also, in a recent study, the use of neuromuscular blockade resulted in decreased inflammatory markers, which was partly attributed to a reduction in epithelial and endothelial injury.42 While the use of neuromuscular blockade should be considered in severely hypoxaemic patients, relevancy of injured lung and increased alveolar stress are important. All these proposed mechanisms also apply to milder patients with lung injury, in whom NIV may be considered as a favourable intervention. The risks of patient self-inflicted lung injury should be weighed against the short and long-term consequences of heavy sedation and paralysis.43
In a study conducted in subjects with acute hypoxaemic respiratory failure, patients failing NIV have been shown to have higher tidal volumes.44 Controlling tidal volume in these patients is almost impossible without high levels of sedation and often paralysis, which is mostly achieved with invasive ventilation.
Respiratory drive is sometimes assessed through respiratory rate, though it is not reliable. A high respiratory drive can be evaluated by oesophageal manometry, diaphragm ultrasound, electrical activity, electromyography, or by asking the patient if they have ‘air hunger’.30
Recently, the ‘mechanical hypothesis’ was put forward.45 In an inhomogeneous lung caused by inflammatory oedema, the forces acting on alveoli with different elasticity can be entirely different and the TPP can be doubled in these injured regions. Decreasing the inhomogeneity can be achieved by two manoeuvres: prone positioning and/or PEEP.46 The former is clearly indicated in moderate-to-severe ARDS, and it is usually performed with IMV. Prone positioning with NIV was applied with success, though it has many limitations and needs specific expertise.47 PEEP may decrease lung inhomogeneity through limiting lung collapse, increasing lung volume and, thus, reducing WOB.48 On the other hand, increasing PEEP also increases the mechanical power applied to the lung parenchyma, facilitating VILI.45 It can also disturb the perfusion. Indeed, it is known that cardiac output and shunt are related; a decrease in cardiac output decreases the shunt.49 An increase in PaO2 but a reduction in SvO2 with PEEP increases shows that the improvement in oxygenation is not related to the recruitment of the collapsed alveoli.50
COMPLICATIONS OF NONINVASIVE VENTILATION
One of the complications of NIV is gastric distention, but it is likely to be overestimated. The tone of upper and lower oesophageal sphincters in a healthy person are 40 cm H2O and 32 cm H2O, respectively.51 The fear of starting NIV after bariatric surgery is justified by gastric distention and anastomose leakage and supported by few case reports.52 In a review which included 11 studies of 5,801 patients undergoing bariatric surgery, 1,293 (22%) of patients received PPV and only 11 cases of anastomose leak were reported, yielding a rate of 0.8%, which was the same as in patients who did not receive PPV.53
Other studies have shown no relation between anastomose leakage and NIV after bariatric surgery in obstructive sleep apnoea syndrome patients.54,55 Nevertheless, sphincters may not function properly in some patients and can be as low as 11 cm H2O.56 Caution to high airway pressure is advocated in these patients.
Skin breakdown is a frequent complication of NIV and has been reported to occur in up to 48% of NIV patients.57 It ranges from transient erythema to skin necrosis and increases a patient’s intolerance to a mask. The use of total face masks or helmets and interchanging between different types of masks are suggested if an NIV session will be long.58
HOW TO UNDERSTAND IF A PATIENT IS FAILING
The intensive care patient population can be heterogeneous, and it is logical to trial NIV in patients with respiratory failure unless a clear contraindication or potential harm is present. Problems associated with patient selection are given in Table 1.
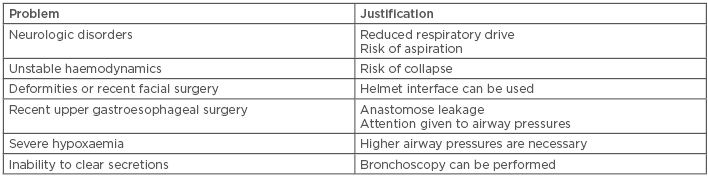
Table 1: Problems associated with patient selection for noninvasive ventilation.
The importance of detecting failing patients cannot be overemphasised because it is shown that delaying IMV increases mortality.22,59,60 Close evaluation is necessary after initiating NIV therapy and laboratory values can also assist managing clinicians. Accessory respiratory muscle involvement, such as the sternocleidomastoid muscle, is associated with increased WOB and respiratory drive, and diminishing activity of the sternocleidomastoid muscle can be used as a surrogate for adequate pressure support level.61 Predictors of failing NIV are summarised in Box 1.41 Several studies have also addressed this situation.62-64 Minimum improvement in PaO2:FiO2 after 1–2 hours of NIV, higher illness severity at admission, and higher respiratory rate were found to be indicators of NIV failure.65
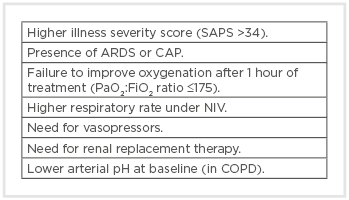
Box 1: Predictors of noninvasive ventilation failure in acute respiratory failure.47
ARDS: acute respiratory distress syndrome; CAP: community-acquired pneumonia; COPD: chronic obstructive pulmonary disease; FiO2: fractional inspired oxygen; NIV: noninvasive ventilation; PaO2: arterial oxygen partial pressure; SAPS: Simplified Acute Physiology Score.
If adequate oxygenation, relief of dyspnoea, or a decrease in respiratory rate cannot be achieved within 1 hour or the neurologic condition deteriorates, proceeding to intubation is necessary. Ventilator asynchrony is another major factor for NIV failure, but it has been shown that a clinician’s ability to recognise this issue is low.66 Meticulous attention to flow and pressure waveforms is required after every change in the ventilator setting to improve patient outcomes. Appropriate interface selection is also crucial because leaks will cause an insensitive trigger, affecting patient tolerance and synchrony.65
CONCLUSION
The relative success of NIV in respiratory failure patients with hypercarbia compared to hypoxaemic patients can be due to the more rapid reversible nature of the hypercapnic disease, such as muscle unloading and changes in the workload of the heart. The more time taken for the reversal in parenchymal infection, inflammation, or ARDS may necessitate more extended ventilatory support and careful observation of the patient. Favourable effects of NIV have been demonstrated in a select group of patients with CPO, patients with acute exacerbations of COPD, postoperative patients with atelectasis, and immunocompromised patients, though some concerns are inherent in most cases. It would be wise to select patients carefully according to observed pathophysiological changes.