Meeting Summary
The third Encuentro Latinoamericano de Infecciones Respiratorias Recurrentes (ELAIR) took place in Mexico City, Mexico, on 11th−12th May 2017. ELAIR brought together experts from across Latin America and further afield, continuing an extraordinary didactic exercise on the cutting-edge advances of respiratory medicine. Impressive progress has been made in the past 15 years, with new treatments available to manage and prevent airway infections. It remains to be seen how this might affect the related conditions of wheezing and asthma in predisposed and sensitised subjects. However, early data suggest that lower respiratory infection rates may reduce the development of the above conditions which are closely related to viral infections. Immunomodulators that both prime the immune system to fight infection and reduce inflammation are likely to play a major role in secondary and even potentially primary prevention of atopic diseases.
ANTIMICROBIAL RESISTANCE: A WORLDWIDE THREAT
The global antimicrobial resistance (AMR) crisis continues to increase in severity. The persistent worsening of the situation in recent years lends a pessimistic perspective to any summary of the state of play. Yet, through this perspective we may gain clarity, allowing the medical community to attack the problem with new ideas and strategies.
There are few better illustrations of the radically altered landscape of infectious disease care than comparing a case study from the beginning of the antibiotic era with another from the present day. In a seminal paper, Abraham et al.1 reported the case of a 4-year-old child who was among the first patients in the world to be treated with penicillin. The patient presented with cavernous sinus thrombosis, secondary to pyoderma of the eyelids, a typical post-measles complication, caused by a bacterial superinfection by Staphylococcus aureus. The infection was refractory to sulfapyridine therapy and, upon admission, the child was semi-comatose, incontinent of urine and faeces, with gross oedema of both eyelids, bilateral proptosis, complete bilateral external ophthalmoplegia, and neck rigidity. He had meningoencephalitis due to the massive staphylococcal infection. Following intravenous (IV) penicillin treatment the child improved rapidly and after 2 weeks his cerebrospinal fluid had become sterile.1
Sixty-seven years later, a 70-year-old man developed mitral endocarditis with a vancomycin-resistant Enterococcus faecium infection following a Whipple procedure to treat a pancreatic tumour. He displayed persistent bacteraemia after 12 days of treatment with linezolid. Therapy was changed to daptomycin (6 mg/kg/48 hours) with no effect; on Day 17 daptomycin dose was increased to 8 mg/kg/48 hours and doxycycline and gentamicin were added. Ten days later blood cultures remained E. faecium-positive (daptomycin minimum inhibitory concentration [MIC]: 2 mg/L). Therapy was changed to quinupristin/dalfopristin (MIC: 0.5 mg/L); however, the patient died on Day 33 of treatment with positive blood cultures.2 Such severe cases of bacterial infection, resistant to all available antimicrobials, can now been found in hospitals across the world.
In 1992, the USA infectious disease specialist Harold Neu, published a paper warning of complacency regarding the threat of AMR.3 Neu pointed out that many bacterial species were now resistant to the vast majority of older antibiotics. He highlighted the overuse of antibiotics, both in hospitals and the wider community, as the source of the problem and called for antibiotic control programmes, improved hygiene, and research to synthesise new agents in order to reduce further resistance and to avert a global AMR crisis. Twenty-five years later, despite the publication of hundreds of research papers on the subject and numerous national initiatives, society has failed to wean itself from irresponsible use of antibiotics and the crisis predicted by Neu has come to fruition. Recently, however, the gravity of the threat has begun to condense local initiatives into global action.
The World Health Organization’s (WHO) 2014 global report on AMR4 is the first attempt to create a worldwide perspective on AMR surveillance. The report identified substantial gaps in surveillance, characterised AMR as a threat to the achievements of modern medicine as a whole, and predicted the arrival of the post-antibiotic era if a significant change in direction is not achieved. The WHO has called for governments to improve surveillance, to allow a full understanding of the extent of AMR as a first step towards tackling the issue. The report stated: “A post-antibiotic era – in which common infections and minor injuries can kill – far from being an apocalyptic fantasy, is instead a very real possibility for the 21st Century.”4 This statement is starkly illustrated by the detection of both Escherichia coli resistant to third-generation cephalosporins or fluoroquinolones and methicillin-resistant Staphylococcus aureus (MRSA) in five of the WHO’s six world regions (the Americas, Europe, Africa, the Eastern Mediterranean, South East Asia, and the Western Pacific) and multiple-drug resistant (MDR) Klebsiella pneumoniae in all world regions.4
A major UK government publication predicted a rise in deaths attributable to AMR from a low estimate of 700,000/year in 2014 to a potential 10 million/year in 2050, if the resistance crisis is not managed; these statistics indicate that AMR-related deaths would eclipse those caused by cancer. This number may appear dramatic, but it is in fact based on resistance of only three organisms (K. pneumonia, E. coli, and S. aureus) suggesting it is a very conservative estimate of the likely impact of unchecked resistance.5
THE EPIDEMIOLOGY OF RESISTANT ORGANISMS
AMR can be found in virtually all known forms of micro-organisms including viruses (Varicella zoster [chicken pox/shingles]), protozoa (Plasmodium sp. [malaria]), fungi, and bacteria. The most important factor determining AMR is the exposure of an organism to the selection pressure of an antimicrobial compound. The current state of AMR has seen MDR move from the hospital to the community, and community-acquired infections by MDR micro-organisms can now be found all over the world.
Focussing on bacterial species, the major organisms of concern in hospitalised patients are MDR pneumococci, MRSA, and vancomycin- resistant enterococci, among Gram-positives. Among Gram-negative bacteria, bacilli expressing extended spectrum β-lactamases (ESBL), carbapenem-resistant Klebsiella sp. and other enterobacteriaceae, MDR Pseudomonas aeruginosa, and MDR Acinetobacter baumannii are of most concern. Gram-negative bacilli represent the organisms of greatest concern due to their battery of defence mechanisms against antimicrobial compounds. MDR Gram-negatives are now found in the community and in animal hosts, causing serious community-acquired as well as hospital infections. The first pan-resistant organisms are members of this group, and are discussed later.
Streptococcus pneumoniae is the major primary pathogen of community-acquired respiratory tract infections (RTI). In a 2014 study from Seoul, South Korea, five strains of pneumococcus were identified which were only susceptible to those antibiotics reserved for highly resistant infections (linezolid, tigecycline, and vancomycin).6 In Mexico, a gradual erosion in the susceptibility of S. pneumoniae to the macrolide class of antibiotics is evident in comparisons of 2005–2007 data with that from 2008–2012. This group of antimicrobials is particularly important for paediatricians and the current prevalence of resistance (30–40%) precludes their use as an empiric therapy in Mexico.7
Enterococci, most commonly Enterococcus faecalis, are becoming increasing prevalent as hospital-acquired infections. In Europe, 90% of E. faecalis isolates are susceptible to vancomycin; however, in Canada, Latin America, and the USA, respective resistance prevalences of 22%, 28%, and 79% have been detected.8
MRSA represents >25% of S. aureus isolates found in the majority of southern European countries (Figure 1). The majority of central and northern European countries have >10% MRSA prevalence, with lower resistance generally restricted to Scandinavian countries and the Netherlands.9
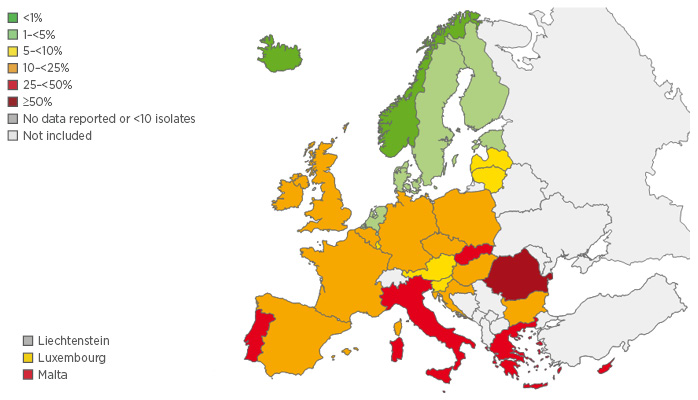
Figure 1: Proportion of MRSA isolates in participating countries in 2013.9
MRSA: methicillin-resistant Staphylococcus aureus.
Enterobacteria, in particular E. coli, are the most common Gram-negative bacteria encountered in hospitals. Antibiotic selection pressure has driven the evolution of hydrolysing β-lactamase enzymes creating ESBL capable of destroying cephalosporins and carbapenems. The worldwide prevalence of ESBL-producing E. coli has been increasing over the last decade, with the highest levels observed in the Asian and Pacific regions (28%) and Latin America (23%), according to 2009−2010 figures.10 In K. pneumoniae, ESBL-producer prevalence has shown a similar upward trend, with 2008−2010 figures breaching 50% within Latin America (53%).10 In the USA, national ESBL-producer prevalence was 12% in a study examining four species of enterobacteriaceae; however, there was significant regional variation with prevalence as high as 23% in the mid-Atlantic region and as low as 4% in the west-north-central region. Additionally, many of the strains expressed more than one gene for ESBL-production conferring multiple resistance pathways.11 Hundreds of β-lactamase variants are now present worldwide, and human carriage of ESBL-producing organisms is increasing globally.12
Despite their relatively protected status, carbapenems have been used liberally in immunosuppressed patients worldwide. In some European countries, such as Italy and Greece, carbapenemase-producing enterobacteriaceae are endemic. Even in the Netherlands which has extremely strict use of antimicrobials and search and destroy programmes targeting resistant organisms, ESBL-resistance cannot be avoided. Data indicate that single hospital outbreaks of carbapenemase-producing enterobacteriaceae have occurred; based on current trends, the situation is likely to worsen.13
Plasmid-mediated colistin resistance (MCR-1) represents the latest emerging threat. The polymixin colistin has been on the market since the 1960s, but has been avoided due to toxicity and poor penetration. Nevertheless, it remains highly active against Gram-negative bacteria and has become the last resort antibiotic against many MDR infections. The extent to which the mcr-1 gene has disseminated among enterobacteriaceae remains to be determined; however, two cases of bacteraemia caused by E. coli producing MCR-1 have been reported in Switzerland. Neither patient had received previous treatment with polymyxins, travelled abroad in the past few years, nor had exposure to farm animals. These data suggest a silent, horizontal spread of the mcr-1 gene plasmid in community-acquired E. coli, with the potential for disastrous consequences.14
In many minds, tuberculosis is an infection associated with the 19th rather than 21st century, yet MDR-tuberculosis, resistant to isoniazid or rifampin, is likely present in every country in the world. Furthermore, many countries have now had at least one case of extensively drug-resistant tuberculosis, which has few treatment options, with poor surveillance likely to be a cause of widespread under-reporting.4
ANTIBIOTIC CONSUMPTION
AMR is an elegant example of natural selection at work. Random mutations conferring resistance are advantageous for bacteria exposed to the selection pressure of naturally produced antimicrobials in the environment. However, this natural selection has been rapidly accelerated by the profligate use of antibiotics during the 20th century. Excessive use is not limited to medicine, it is a major issue in meat production, leading to direct resistance as well as downstream consumption by humans. Inappropriate use extends beyond use in non-bacterial infections, to inadequate dosing or treatment durations which favour survival of resistant bacteria.
Antimicrobial use is common outside human medicine, in fact approximately 80% of the annual use of antimicrobials in the USA occurs in livestock, agriculture, and aquaculture.15 China uses more antibiotics than any other country; a 2007 survey estimated that 210 million kg of antibiotics were used, totalling >46% of these drugs used in farming.16 China, alongside India, is the top consumer of antimicrobials and, therefore, the top producer of MDR organisms. Data on ESBL-producing E. coli in the faeces of hens, pigs, and cattle from Hong Kong shows a steady rise in all three species. In 2013, 70–80% of pigs and hens, and approximately 45% of cows tested had resistant gut flora.17 There are no studies illustrating these relationships directly; however, there is an intuitive understanding of the relationship between the consumption of antimicrobial-treated animal products and AMR in humans.
Globally, consumption of antimicrobials rose by 40% between 2000−2010; however, this pattern hides a varied picture of reduced consumption in some countries and large increases in others. Annual consumption varies by a factor of ten across all middle and high-income countries. The economies of Brazil, Russia, India, and China, along with South Africa, were responsible for the vast majority of the growth from 2000−2010.18
The relationship between direct human antimicrobial consumption and AMR has been shown in Europe, where a surveillance system exists. A large range of consumption exists from approximately 10 defined daily dose per 1,000 inhabitants per day in countries such as Estonia and the Netherlands, and close to 40 defined daily dose per 1,000 inhabitants per day in Greece.19,20 The countries consuming high amounts of antibiotics, such as Greece and Italy, unsurprisingly, tend to have the highest prevalence of resistance.
RESISTANCE IN PNEUMOCOCCI
We define a micro-organism’s resistance to a specific antimicrobial based on the MIC, the lowest concentration that visibly inhibits bacterial growth overnight. MIC are subject to change overtime, and are calculated and released by specific organisations in Europe, including the European Society of Clinical Microbiology and Infectious Diseases (ESCMID), and the Clinical and Laboratory Standards Institute (CSLI) in the USA.
S. pneumoniae is the most common cause of bacterially acquired RTI. In Mexico, the susceptibility of S. pneumoniae to the antibiotics tested decreased when comparing 2005–2007 and 2008–2012 data from the large T.E.S.T trial.7 In many cases, when a bacterial strain develops resistance to a single antibiotic within a class, for example through β-lactamase production, the mechanism will confer resistance to all antibiotics within this class.
Common treatment options for community-acquired RTI include trimethoprim-sulfamethoxazole, tetracyclines, chloramphenicol, macrolides/azalides/ ketolides, β-lactams, and fluoroquinolones. The macrolides, β-lactams and fluoroquinolones are perhaps the most commonly utilised and the consequent resistance mechanisms are well understood.
S. pneumoniae: Macrolide Resistance
Macrolides have been available since the 1950s, and have been frequently used against pneumococci, particularly in the paediatric population due to their safety and efficacy profile. Macrolides are protein synthesis inhibitors, targeting both peptidyltransferase and ribosomal translation. Hence, the macrolide must achieve a cytoplasmic concentration sufficient to have an effect, and pneumococci can become resistant by expressing efflux pumps (mefA or mefE genes) to extrude the antibiotic. A second, more complex and efficient resistance mechanism involves ribosomal methylase (ermB or, rarely, the ermA gene), which dimethylates pneumococcal 23S ribosomal RNA, preventing access to the antibiotic binding site. Other mutations that affect macrolide binding related to ribosomal proteins L4 and L22 have been described.
Pneumococcal infections have demonstrated the ability to acquire resistance in vivo. A 2002 published letter offers a tragic example of treatment-emergent macrolide resistance. A 28-year-old man presented with a 5-day history of cough and dyspnoea; sputum culture revealed S. pneumoniae infection. Treatment with 500 mg/day IV of azithromycin was initiated and the patient responded well; however, at Day 4 he began displaying progressive respiratory insufficiency and was transferred to intensive care. Despite administration of ceftriaxone and vancomycin, the patient progressed to multi-organ failure and died. Isolates of the S. pneumoniae taken after the patient’s deterioration, displayed the same genetic markers (BOX typing patterns) as the initial culture, indicating it was the same strain. However, while initial isolates showed full susceptibility to all antibiotics tested, including penicillin, the second isolate had become resistant to erythromycin, azithromycin, and quinupristin/dalfopristin. Sequencing revealed that resistance was conferred by a mutation of the ribosomal protein L22.21
Such cases are not isolated. In northern Africa, trachoma is an endemic and a major cause of sight loss during childhood. The WHO conducts programmes in the region aimed at preventing trachoma via a single dose of azithromycin. During one such programme in Morocco, a 2.5-month- old infant with nasopharyngeal carriage of S. pneumoniae (serotype 22F) developed resistance after a single dose of the macrolide. In this case, resistance was conferred by a 23S ribosomal RNA 2059A>G mutation resulting in a MIC change from 0.5 mg/L pre-mutation to >256 mg/L post-mutation.22
Macrolide resistance is increasing worldwide, and is often associated with penicillin resistance. Two recent studies indicate that in vitro resistance, at any level, is a predictor of treatment failure. The development of treatment-emergent resistance is not an isolated event; therefore, care must be taken when prescribing a single macrolide to patients with extrameningeal pneumococcal infections. The prevalence of resistance will dictate the need to reassess current recommendations for the treatment of community-acquired infection.
S. pneumoniae: β-lactam Resistance
S. pneumoniae is a resident of the nasopharynx found exclusively in humans. The bacterium acquires its β-lactam resistance via transformation of foreign genetic material from other streptococci. The transfer of genetic material to the genes coding for penicillin binding proteins, reduces their binding affinity for the β-lactams, thereby decreasing the activity of these compounds. Unlike other resistance mechanisms, such as acquisition of a hydrolysing enzyme, changes in penicillin binding proteins confer gradual increases in resistance as the antibiotic’s ability to bind and block bacterial-wall formation is degraded by gradually acquired genetic changes.
With no animal reservoir, eradication of S. pneumoniae would seem to be a possibility. However, the existence of 93 S. pneumoniae serotypes, its ability to transit rapidly between individuals, and its capacity to confer AMR via gene transformation make S. pneumoniae an extremely adaptable organism. These features make areas of high-density human interaction, such as daycare centres, into factories for AMR with children swapping serotypes and bacteria, in turn, trading in resistance genes.
β-lactams act in a time-dependent fashion. The major pharmacokinetic/pharmacodynamic parameter which predicts eradication is the time that the concentration of the antibiotic in serum is above the MIC. Hence, dosing intervals are more important than increased dose in improving eradication. For example, during the standardisation of amoxicillin/clavulanate therapy increases in amoxicillin dose from 500 mg to 875 mg resulted in an increase in the time above the MIC from 36% to 44%, while a change from twice-daily to three-times-daily dosing increased the time above MIC from 36% to 55%.
The choice of β-lactam antibiotic is also an important consideration, particularly in the paediatric population. Among the oral cephalosporins, cefuroxime and cefpodoxime have the greatest activity against penicillin-susceptible S. pneumoniae. However, they have MIC that are 1–4 dilutions higher than amoxicillin against non-susceptible strains and cause more collateral damage than penicillins. As a result, when treating a pneumococcal respiratory infection orally, amoxicillin remains the β-lactam with the best pharmacokinetic and pharmacodynamic relationship, particularly in children, and the lowest impact on the resident flora. The oral cephalosporins, cefixime, cefaclor, ceftibuten, and loracarbef, and macrolides generally are not recommended because of the decreased activity of these agents against non-susceptible pneumococci.23-25
Even in penicillin-resistant strains, adequate dosing of the correct β-lactam remains an effective therapy. There have been no documented treatment failures with adequate dosing of penicillins or third-generation cephalosporins in patients with extrameningeal infections.26 There is no evidence of increased mortality in penicillin-resistant pneumonia when adjusted for severity of disease and comorbidity. However, increased mortality is reported in meningitis caused by penicillin non-susceptible strains.
S. pneumoniae: Fluoroquinolone Resistance
Fluoroquinolones became part of the pneumococci treatment arsenal in the 1980s with the introduction of the relatively inactive ciprofloxacin. A large number of potential subsequent fluoroquinolone compounds failed due to toxicity concerns. However, those currently licenced, including levofloxacin and moxifloxacin, have excellent activity against pneumococcus.
The target of fluoroquinolones are the enzymes DNA gyrase and topoisomerase IV, which control the winding and unwinding of the bacterial genome required for replication. Pneumococci have several resistance mechanisms against fluoroquinolones, the most common involve mutations in genes encoding the target enzymes. Mutations frequently occur in parC, which encodes the A subunit of DNA topoisomerase IV, or gyrA, which encodes the A subunit of DNA gyrase. Less frequently, mutations can be found in the genes parE and gyrB, encoding the B subunits of these proteins. Efflux pumps also play a role in decreasing susceptibility, though these are less significant in conferring resistance. Acquisition of both parC and gyrA mutations confers complete fluoroquinolone resistance.27,28
The possibility of S. pneumoniae strains possessing an occult resistance mechanism, due to the presence of either a parC or gyrA mutation, creates potential issues for fluoroquinolone therapy. Indeed, as with macrolide therapy, there are well-documented examples of treatment- emergent resistance during fluoroquinolone therapy.29-32 In all currently documented cases, the parC or gyrA paring has been detected and infections have displayed a staggering level of resistance to fluoroquinolone therapy.
Treatment-emergent resistance is more common in immunocompromised patients, due to reduced immune response, increased length of carriage, and increased density of organisms. Patients with myeloma, for example, may have greater bacterial diversity than otherwise healthy patients with pneumonia. Patients with structural lung disease are also at increased risk of treatment-emergent fluoroquinolone resistance.
Given the risk of occult single-gene mutations, it may be prudent to avoid the use of fluoroquinolone monotherapy in patients treated with fluoroquinolones during previous months. Furthermore, in patients with severe documented pneumococcal infection, caused by strains with levofloxacin MIC ≥1 µg/mL, fluoroquinolones should be avoided or used in combination.29,30,32,33
STAPHYLOCOCCUS AUREUS
MRSA is perhaps the best-known MDR strain. Methicillin was the first synthetic β-lactam synthesised in response to penicillin resistance in 1959. A year later, the first MRSA isolates were cultured in UK laboratories. Hospital-acquired MRSA infection was detected in Western Europe, the USA, and Australia in the following decade. By the 1980s MRSA was making headlines outside the medical community and community-acquired MRSA had been documented. The end of the 1990s saw the first appearance of vancomycin-resistant MRSA;34 now, MRSA represents a global community and hospital epidemic. In the T.E.S.T survey, the prevalence of MRSA in Mexico was 45%.7
The treatment of MRSA infections has traditionally relied on glycopeptides, mainly vancomycin; however, there has been a progressive erosion of the MIC of vancomycin, and alternative therapies are becoming important. Daptomycin is the best-known alternative, but has some limitations including being inadequate for pulmonary infections due to neutralisation by pulmonary surfactant, and bacteria infecting other locations beginning to show decreased susceptibility. Linezolid is another useful antibiotic in patients with serious MRSA infections that cannot be treated with glycopeptides; however, bone marrow toxicity limits its use and plasmid-mediated resistance represents a potential future threat.
Outcomes for methicillin-sensitive S. aureus, treated with vancomycin, are significantly worse than for MRSA infections.35 Other issues with vancomycin therapy include poor tissue penetration, a slow bactericidal activity, difficulty in optimising dose, MIC creep, and the emergence of strains with varying levels of vancomycin susceptibility, including heteroresistant S. aureus, intermediate-level resistant S. aureus, and vancomycin-resistant S. aureus.36 Some new agents for MDR Gram-positive cocci, such as MRSA, include two highly active lipoglycopeptides, oritavancin, and dalbavancin, oxazolidinone tedizolid, and two new cephalosporin β-lactams ceftobiprole and ceftaroline.
GRAM-NEGATIVE BACTERIA
Gram-negative bacteria are more complex than their Gram-positive counterparts and have an extraordinary capacity for defence and plasticity against antimicrobial compounds. Mechanisms include loss of porins, which reduces the penetration of some antibiotics though the cell membrane, conferring resistance to β-lactams such as imipenem; production of β-lactamases which hydrolyse antibiotics in the periplasmic space; over-expression of transmembrane efflux pumps, which target β-lactams, quinolones, aminoglycosides, tetracycline antibiotics, and chloramphenicol; metabolic bypass mechanisms, usually through a new enzyme bypassing the pathway inhibited by the antibiotic; ribosomal mutation or modification which impedes the binding of some tetracycline and aminoglycoside antibiotics; target-site mutations affecting the quinolones by altering DNA gyrase and topoisomerase IV; and enzymes that directly modify and inactivate antibiotics like aminoglycosides and ciprofloxacin.37 Antibiotic selection pressure has driven the incidence of mutations, leading to resistance mechanisms following the introduction of each new class of antibiotic from ampicillin in the 1960s (TEM, SHV serine, β-lactamases), the cephalosporins in the 1980s (AcrAB, blaSHV, blaTEM, AmpC-type, β-lactamases), and carbapenems from the 1990–2000s (CTX-M-15, VIM, IMP, NDM-1, KPC, OXA-48, Porin defects) (Table 1).38-41
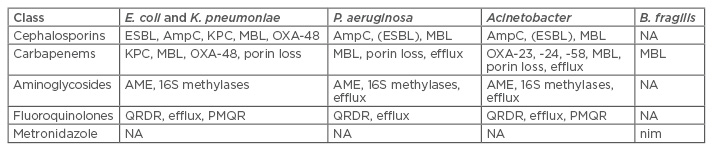
Table 1: Gram-negative resistance mechanisms.
AME: aminoglycoside modifying enzymes; B. fragilis: Bacteroides fragilis; E. coli: Escherichia coli; ESBL: extended spectrum β-lactamase; K. pneumoniae: Klebsiella pneumoniae; KPC: K. pneumoniae carbapenemase; MBL: metallo β-lactamase; NA: not available; P. aeruginosa: Pseudomonas aeruginosa; PMQR: plasmid-mediated quinolone resistance; QRDR: quinolone resistance-determining region.
The β-lactamases of enterobacteriaceae, such as E. coli, have been evolving for more than 50 years. Four classes of β-lactamases are recognised: Class A, which can confer resistance to many β-lactams through the production of ESBL and carbapenemases, such as the K. pneumoniae carbapenemases that have become widespread in many parts of the world; Class B, metallo-β-lactamases, which are carbapenemases; Class C, cephalosporinases; and Class D, oxacillinases.
The terminology used to describe resistance has been evolving to keep pace with the extent of the resistance crisis. Researchers and physicians have reclassified organisms from resistant, to MDR, to extensively drug–resistant and, finally, to pan drug–resistant, for those bacteria with resistance to all licenced antimicrobials.42
Severe E. coli infections before the antibiotic era would be susceptible to 13 classes of antibiotic available today. The emergence of the ESBL-producing enteric bacteria, following selection by those same antibiotics, leaves only four parenteral treatment options in high resistance areas (tigecycline, carbapenems, amikacin, and colistin), most of which represent a single antibiotic rather than a class; however, all these options have limitations. Plasmid-mediated resistance to polymyxins like colistin is the latest threat and toxicity of aminoglycosides has further reduced the number of available antibiotics to combat resistant organisms. This has resulted in increased use of carbapenems with the inevitable consequence of a progressive rise in resistance to these agents.
Carbapenemases are now emerging in various forms within Gram-negative bacteria. K. pneumoniae carbapenemases may be found not only in K. pneumoniae but also other enteric bacteria. Metallo-β-lactamases are produced by both P. aeruginosa and enterics. Oxacillinases capable of inactivating carbapenems are also emerging, both in Acinetobacter, and in both K. pneumoniae and E. coli.
COMBATTING ANTIMICROBIAL RESISTANCE
Little progress has been made in tackling resistance since the predictions of Harold Neu, some 25 years ago. Improved surveillance and the development of improved diagnostic tests are necessary to begin to address increased AMR. Molecular diagnostic testing creating a single point-of-care blood test to identify bacterial versus viral infections will help in rationalising antibiotic use. Pressure from an increasingly aware public, and consequently increased political pressure, is leading to more robust efforts against the spread of MDR bacteria and attempts to slow the emergence of resistance through improved antibiotic stewardship. Key to both these approaches is the prevention of infection. The increased understanding of AMR outside the medical community is accelerating research into new drugs through increased collaboration between drug companies, government, and non-profit organisations. Similarly, improved global co-ordination supported by organisations, such as the WHO, will help to combat highly mobile resistant pathogens.43
APPROPRIATE TREATMENT OF COMMUNITY-ACQUIRED PNEUMONIA: LESSONS FROM A CASE STUDY
A 78-year-old woman was admitted to hospital. She had been symptom-free the previous evening before the rapid onset of chills, fever (oral temperature: 39.5°C), right-sided pleuritic chest pain, mild non-productive cough, and dyspnoea associated with minimal physical exertion. The patient had a history of rheumatoid arthritis, treated with prednisone (5 mg/day) during the previous 3 months; mild hypertension controlled with hydrochlorothiazide; and hospitalisation for acute pyelonephritis 2 months previously, during which she received fluoroquinolone treatment. She was living alone at the time of hospitalisation.
Physical examination revealed the patient was lucid and responsive. She displayed tachypnea (respiration rate: 35/min), tachycardia (pulse rate: 120/min), and her blood pressure was 130/85 mmHg. The patient appeared hydrated with no peripheral adenopathy. Pulmonary examination revealed signs of consolidation and crackling rales in the right hemithorax. Heart rhythm was regular with no murmurs. There were no signs of jugular engorgement or peripheral oedema. Her abdomen was soft and without organomegalies; her neurological exam was normal.
Subsequent chest X-ray showed a well-defined infiltrate typical of pneumonia. The extent of the infection, also assessed by computed tomography scan, was large enough to touch the pleura, explaining the pleuritic chest pain.
Diagnostic Lessons
Chest X-ray is the essential diagnostic tool for acute pneumonia. As well as confirming the diagnosis, it may indicate the presence of complications (effusion, abscess, tumour, etc.), provide possible evidence of aetiology, and allow tracking of disease progress.
Along with chest X-ray, blood cultures are the second essential diagnostic tool, though not in the immediate phase due to the delay in receiving results. Blood cultures confirming bacterial infection are almost exclusively positive for pneumococcus in patients without risk factors for Gram-negative bacillus infection. In those with risk factors, E. coli is the second most common micro-organism found. However, other pathogens must also be considered. The abrupt onset and early signs of consolidation in the patient suggest that Legionella pneumophila is an unlikely candidate. An opportunistic infection, such as Pneumocystis jiroveci, is improbable due to the sharply defined radiological fissure (‘ground glass’ presentation is more typical), unilaterality, and low doses of prednisone which would be unlikely to cause significant immunosuppression.
S. pneumoniae is the most likely candidate micro-organism. The patient’s age, recent hospitalisation, and pretreatment with a fluoroquinolone (ciprofloxacin), should alert physicians to the possibility of a MDR pneumococcal infection. Turning to Gram-negative bacillus the likelihood of E. coli infection is increased by the presence of risk factors including female gender, age, and recent hospitalisation.
Treatment Lessons
The patient’s age, per se, is not a risk factor for a complicated disease course. However, the combination of age and comorbidities with possible immunosuppression do represent risk factors for a complicated course and unusual aetiologies of community-acquired pneumonia. In addition, tachypnea is a serious physical sign of prognostic importance.
Acute pneumonia requires empirical antibiotic therapy. Azithromycin is a poor choice of antibiotic in this case as pneumococcal infections are frequently resistant to macrolides and azithromycin is not active against Gram-negative infections, which remain a possibility in this case. Amoxicillin has little effect against most Gram-negative bacteria of interest in community-acquired pneumonia. Ceftriaxone is a serious consideration, because the resistance of pneumococci to the cephalosporins is very low, and ceftriaxone is also active in the majority of Gram-negative bacteria of interest in the community-acquired pneumonia. However, it does not cover Legionella, or other ‘atypical’ organisms. A fluoroquinolone would be a good choice if the previous treatment with ciprofloxacin and resultant risk of treatment-emergent resistance in pneumococci did not contraindicate its empirical use. Combined ceftriaxone-azithromycin therapy is the best choice for empiric treatment of serious community-acquired pneumonia as it covers the vast majority of potential pathogens. This combination has been shown more effective than monotherapy with a β-lactam in some patients with pneumococcal pneumonia.
Treatment Response
The patient received the combination of IV ceftriaxone-azithromycin, and after 48 hours showed improvement, with a temperature of 37.8°C and a reduced feeling of pain. Blood culture results revealed S. pneumoniae with intermediate resistance to penicillin (MIC: 1 mg/L). The results allowed the attending physicians to simplify the treatment, changing to an oral route and choosing a classical antibiotic in order to reduce costs.
Switching to an oral schedule is desirable when the patient shows a substantial improvement as it reduces the risk of catheter infection and venous thrombosis, as well as facilitating a move to ambulatory care. In this case, monotherapy with amoxicillin was feasible because the degree of resistance to penicillin is easily overcome with the appropriate dose (1 gram/every 8 hours).