Abstract
Chronic inflammation of skeletal muscle tissues, termed myositis, is associated with inherited muscular dystrophy and acquired inflammatory myopathy. In this review, the role of the organic osmolytes taurine, betaine, myo-inositol, and sorbitol in skeletal muscles in general, and in myositis in particular, is discussed. Evidence indicates that regulated osmolyte pathway activation contributes to normal muscle functioning, which becomes further activated in myositis as part of the tissue’s programme of damage control. On the one hand, osmolytes seem to act as protein stabilisers in muscle fibres counteracting tissue injury but, on the other hand, these compounds also regulate immune cell function. The possibilities for treating myositis through boosting of beneficial or targeting of adversary effects are explored.
THE MULTIPLE FACES OF MYOSITIS
Chronic inflammation of skeletal muscle tissues, termed myositis, can have various origins. It can result from infection, tissue damage caused by inherited diseases, or an acquired autoimmune disease. In the muscular dystrophies, muscle inflammation is secondary, yet it represents a hefty pathogenic factor that contributes to deterioration of the muscle tissue’s integrity. The most common type is Duchenne muscular dystrophy (DMD); other subtypes include Becker muscular dystrophy, facioscapulohumeral muscular dystrophy, and myotonic dystrophy.1 DMD is a severe, and still incurable, X-linked muscle disease caused by protein-disruptive mutations in the dystrophin gene. The absence of dystrophin leads to increased vulnerability to contraction-induced sarcolemmal damage, resulting in cycles of muscle fibre necrosis and failing regeneration. Necrotising myofibres are attacked by macrophages; a few T cells, B cells, and dendritic cells are also found within the inflammatory areas.2 The build-up of inflammation is complexly regulated by an interplay of soluble factors and adhesion molecules. Chemotactic cytokines, termed chemokines, are key players in the inflammatory response associated with DMD, as these diffusible proteins orchestrate the activation and directed migration of leukocytes.3 Our understanding of DMD disease progression has benefited from studies in the murine mdx model, though considerable differences exist between animal and human diseases.4
The idiopathic inflammatory myopathies on the other hand are autoimmune muscle diseases and comprise four main entities: dermatomyositis (DM), polymyositis (PM), sporadic inclusion body myositis (IBM), and immune-mediated necrotising myopathy (IMNM). These different disease subgroups present with distinct clinical and myopathological characteristics, with either blood vessels or muscle fibres acting as the primary immune target. In DM, complement-mediated blood vessel destruction and perifascicular muscle fibre damage and inflammation develop. PM and IBM are characterised by invasion of non-necrotic muscle fibres by auto-aggressive cytotoxic T cells and macrophages, and inflammation builds up mostly at endomysial sites.5 In IBM muscle fibres, additional degenerative phenomena occur, with rimmed vacuoles and inclusions that contain aggregates of ectopic proteins.6 IMNM is an increasingly recognised autoimmune myopathy in subgroups of patients triggered by statin use and associated with autoantibodies directed against 3-hydroxy-3-methylglutaryl-coenzyme A reductase,7 or associated with anti-signal recognition particle autoantibodies.8
THE OSMOLYTE PATHWAY AS A PROTECTOR OF MUSCLE FUNCTION
To anticipate perturbations in volume and osmotic imbalance, cells possess a variety of channels and transporters that enable them to accumulate or release osmotically active substances. In addition to inorganic ions, cells can count on a wide range of organic osmolytes, which include free amino acids and their derivatives, methyl ammonium compounds, and polyols. The intracellular osmolytic pool generally consists of a complex mixture of compounds and, in response to stress, pathway members become activated and complexly regulate each other’s activities.9 The transient regulation of osmolyte transporters in response to changing osmotic conditions stabilises intracellular protein function. Regulation occurs both at the transcriptional and translational level, and on a relatively short time-scale (within minutes).10 The central regulator of the expression of osmolyte pathway genes is the transcription factor tonicity enhancer binding protein, also called nuclear factor of activated T cells 5 (NFAT5).11 Among the NFAT5 target genes are the organic osmolyte carriers for taurine, betaine, myo-inositol, and the enzyme that catalyses sorbitol synthesis. This osmo-protective mechanism represents a universal system in mammalian cells ubiquitously expressed in human tissues.
The muscle is a highly adaptive tissue, capable of increasing its mass in response to exercise, and of restoring damage caused by injury. These processes require hypertrophy and regeneration, respectively, and for that purpose, resident muscle precursor cells, termed satellite cells, are present within the tissue in a quiescent state. Myogenesis is a highly regulated process co-ordinated by regulatory factors both in favour and opposed to differentiation, which become active in an orderly and sequential fashion.12 Interestingly, the NFAT5 pathway is an important regulator of the differentiation of immature myoblasts to mature multinucleate myotubes,13 with NFAT5 levels increasing in the regenerating fibres of mice exposed to experimental muscle tissue injury.14 The important role played by osmolytes in muscle functioning has been made clear in taurine transporter knockout mice, which display muscle fibre atrophy and necrosis, and reduced exercise endurance.15,16 It is well known that in DMD muscle the osmotic balance is perturbed, probably due mostly to passive efflux of osmolytes through the leaky plasma membranes,17 and recent evidence points to an involvement of the osmolyte pathway, also in inflammatory myopathies.
THE OSMOLYTE PATHWAY ASA REGULATOR OF MYOSITIS
In addition to an involvement in damage control and in tissue regeneration, osmolytes have been shown to be potent immune regulators. Both hyperosmotic and inflammatory conditions are able to induce NFAT5 expression and activation in muscle cells in vitro.18 This illustrates how the NFAT5 pathway acts as a more general stress-induced mechanism, equally involved in the muscle tissue’s responses to hypertonicity and to inflammation. In addition, the NFAT5 pathway has been firmly linked to nuclear factor κB (NFκB) activity and subsequent pro-inflammatory gene expression.19 Both the osmolyte pathway members and NFκB p65 subunit protein levels are increased in muscle from DMD and PM/IBM patients.20 In addition, NFAT5 is expressed in inflammatory cells recruited to sites of tissue injury.21 Osmolytes are involved in immune cell function, regulating cell volume as an important aspect of phagocytic activity. Taurine is the predominant free amino acid in granulocytes and in lymphocytes.22 Betaine23 and myo-inositol24 accumulation and transporter expression are potent regulators of phagocytosis in liver macrophages, instigated by NFAT5 activation.25
TAURINE
The semi-essential amino acid 2-aminoethane sulphonic acid (taurine) is the most abundant free amino acid in human tissues. It can either be synthesised in the liver from cysteine by cysteine deoxygenase and cysteine sulphinate decarboxylase, or imported in the cell through its sodium and chloride ion-dependent taurine transporter SLC6A6. In addition to its role as an osmolyte, taurine possesses antioxidant and anti-inflammatory properties, and is important for skeletal muscle function and exercise capacity.15 It has been used as a supplement in energy drinks for athletes for some time. In subjects in their 50s, a multi-nutrient supplement containing taurine has been reported to improve physical function and reduce the inflammation marker interleukin (IL)-6.26 A cell organelle protective effect has also been observed, preserving mitochondrial function27 and counteracting endoplasmic reticulum stress.28 Taurine appears to possess anti-ageing effects, protecting muscle fibres specifically against ageing-associated damage. The content of amino acids in the skeletal muscle alters with age, with taurine levels decreasing, and SLC6A6 knockout mice display accelerated senescence.29
Dystrophin deficiency perturbs taurine metabolism in the muscle, and vice versa, SLC6A6 knockout mice display pathological changes that mimic those observed in the mdx disease model. In mdx mice, taurine content fluctuates in relation to the disease phase. At the onset of the active dystrophic phase at age 4 weeks, reduced taurine and SLC6A6 levels are present in muscle. This deficiency diminishes as the disease progresses to the stable pathology in adult mice.30 In contrast, the canine golden retriever MD model shows upregulated taurine and SLC6A6 levels at age 8 months.31 Thus, differing results have been obtained in different disease models, which fits with the known interspecies variance of dystrophin deficiency characteristics. In patients with active inflammatory myopathy, muscle taurine levels are significantly reduced.32 Interestingly, urinary taurine levels are increased in DM/PM33 and in DMD patients34 compared to healthy controls, suggestive of deregulation of the plasma/tissue taurine balance. For the taurine transporter SLC6A6, different results have also been reported, from lower levels in 3–6-week old mdx compared to control mice,30 to unchanged levels.35 SLC6A6 protein expression is induced in muscle biopsies from inflammatory myopathy patients, mostly in the regenerating and atrophic muscle fibres, notably also in the perifascicular atrophic fibres of DM muscle.20
BETAINE
N,N,N-trimethylglycine (betaine) is a naturally occurring small amino acid derivative. The two main physiological roles of betaine are as an osmolyte to regulate cellular tonicity, and as a methyl donor participating in the control of cellular activities and differentiation. Betaine can be synthesised by the cell through oxidation of choline-containing compounds, or imported from the extracellular matrix by the betaine-gamma-aminobutyric acid (GABA) transporter termed SLC6A12. Betaine has been shown to promote myotube differentiation and hypertrophy in vitro, via insulin growth factor 1-signalling.36 In addition to a beneficial effect on muscle regeneration, betaine could also counteract inflammation, as has been demonstrated by its inhibitory effect on hypoxia-induced adipokine expression.37 Based upon these observations, it can be concluded that betaine could potentially enhance exercise performance, reduce fatigue, and improve muscle function.
Urinary betaine33 and betaine/creatinine ratios38 are higher in DM/PM patients than in controls. These findings parallel reports of elevated taurine, and further corroborate the possible existence of a general osmolyte plasma/tissue imbalance in these patients. While absent from healthy muscle, our immunofluorescence studies show strong staining of the transporter SLC6A12 on a subset of muscle fibres in DMD, DM (Figure 1), PM, IBM, and IMNM tissues, most of which are small atrophic or regenerating muscle fibres.
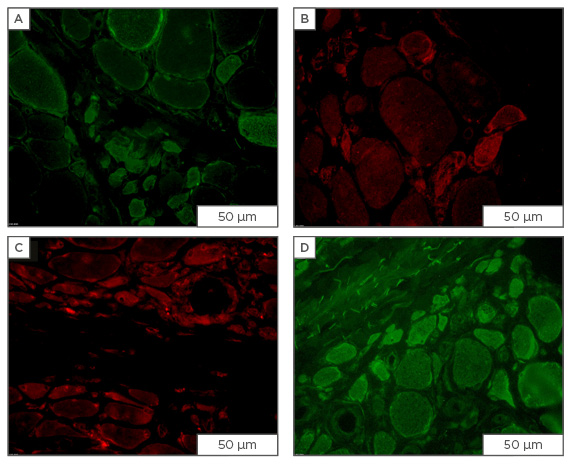
Figure 1: Immunofluorescent staining for osmolyte accumulators in muscle sections from patients diagnosed with dermatomyositis.
Staining for osmolyte accumulators is induced/increased in subsets of muscle fibres of which most are of small-width: A) mouse monoclonal IgG1 anti-SLC6A6 (Santa Cruz Biotechnology, Dallas, Texas, USA; 4 μg/mL); B) mouse monoclonal IgG2b anti-SLC6A12 (Santa Cruz Biotechnology; 4 μg/mL); C) rabbit polyclonal anti-SLC5A3 (Novus, Abingdon, UK; 4 μg/mL); D) goat polyclonal anti-AKR1B1 (Santa Cruz Biotechnology; 1 μg/mL), visualised with secondary antibodies labelled with either AlexaFluor488 (green) or CY3 (red) (Jackson ImmunoResearch Laboratories, Newmarket, UK). Scale bar=50 μm.
Ig: immunoglobulin; AKR1B1: aldo-keto reductase family member B.
MYO-INOSITOL
The essential nutrient cis-1,2,3,5-trans-4,6-hexahydrocyclohexaan (myo-inositol) is a cyclic polyol and is one of the most abundant small organic osmolytes. It regulates different metabolic pathways, in addition to being a key component in preserving the cell’s osmotic balance. Myo-inositol can be synthesised by the cell, or accumulated from the extracellular space. Biosynthesis of myo-inositol starts with the conversion of D-glucose-6-phosphate to L-inositol-1-phosphate in a reaction catalysed by myo-inositol phosphate synthase. In addition to synthesis, import from the extracellular matrix is achieved by the sodium myo-inositol co-transporter SLC5A3. Hypertonic stress conditions lead to the upregulation of SLC5A3 gene expression,39 as well as to the displacement of the transporter to the plasma membrane.10
The SLC5A3 protein is not present in control muscle material but can readily be detected in the majority of biopsy samples from myositis patients. The transporter is expressed on a subset of muscle fibres but, in addition, SLC5A3 expression has been shown on macrophages and T cells infiltrating DMD, DM, PM, and IBM muscle.20
SORBITOL
The sugar alcohol (2R,3S,4S,5S)-hexane-1,2,3,4,5,6-hexol (sorbitol) is synthesised within the cell. The aldo-keto reductase family member B (AKR1B1), also termed aldose reductase, catalyses the reduction of aldehydes and aldehyde forms of glucose. This process makes up the first and rate-limiting step of the polyol pathway of glucose metabolism, reducing glucose to sorbitol. AKR1B1 is expressed in high amounts in normal skeletal muscle, displaying further injury-induced expression.40
Sorbitol accumulation is postulated to play a role in skeletal muscle dysfunction associated with diabetes. In rats subjected to streptozotocin-induced diabetes, muscle sorbitol levels are increased, an effect that can be significantly lowered by administering insulin.41 Insulin decreases intracellular sorbitol by deviating glucose away from the polyol pathway and metabolising it through non-polyol metabolic pathways.42 When an AKR1B1 inhibitor was given to diabetic rats, skeletal muscle sorbitol levels decreased and muscle contractile properties improved.43
Normal skeletal muscle contains high levels of AKR1B1, and in DMD myotubes in vitro, AKR1B1 levels are high and are not further increased by high salt conditions. AKR1B1 levels are increased in the affected muscle fibres of myositis patients, and protein levels are significantly higher in DMD and PM/IBM muscle protein samples compared to control samples.20
TREATING MYOSITIS VIA OSMOLYTE PATHWAY INTERVENTION
Evidence is still sparse but clearly points to osmolyte pathway dysregulation in the muscle tissue of patients suffering from myositis (Table 1). An attractive strategy for treating diseases characterised by osmotic disturbances would be to administer positive osmolytes as a nutraceutical supplement. Such supplements are readily available, are relatively cheap, and only have minimal side effects. Based upon the available scientific evidence, two potential compounds come forward for myositis, in particular, taurine and betaine. Intriguingly, glucocorticoids, the most used pharmacological treatment for DM and PM, but also the most common supportive treatment for DMD, have been shown to increase muscle taurine content.44 In the animal model, the positive effect of glucocorticoids on mdx muscle function can be even further enhanced by administering taurine, with the two drugs exhibiting synergistic therapeutic actions.45 A beneficial effect of taurine supplements has been firmly shown in mdx mice, increasing the muscle taurine content, improving muscle function, and reducing muscle inflammation.35,46 Taurine supplementation represents an attractive approach, as it can conveniently be administered orally and presents no serious adverse effects.47 However, as amino acid patterns of skeletal muscle and blood are age-dependent, with taurine levels increasing from birth to age 15 years,48 the patients’ age may be expected to influence the therapeutic outcome and optimal dosage. The benefits of betaine supplements on muscle endurance and performance49,50 have also been reported, but another study reported no effect of supplementation in healthy adults.51
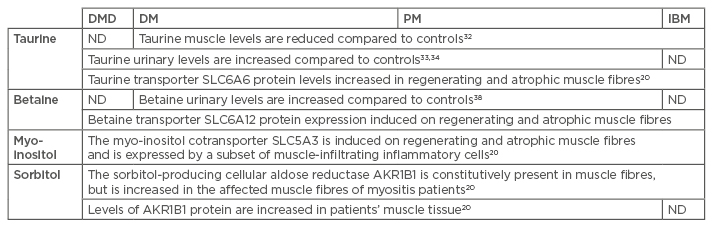
Table 1: Osmolytes in patients diagnosed with myositis: summary of the available data.
DMD: Duchenne muscular dystrophy; DM: dermatomyositis; PM: polymyositis, IBM: sporadic inclusion body myositis; ND: not determined.
Reports on the effects of taurine or betaine supplementation in myositis patients have not become available yet. As the upregulation of osmolyte accumulators in regenerating muscle fibres is a general feature of inflammatory myopathy as part of the re-kindling of myogenetic processes to restore muscle damage,20 osmolyte supplementation could be an amenable supportive therapeutic approach. It should be noted, however, that in DM, osmolyte accumulators are also induced in the perifascicular atrophic muscle fibres, pointing to a possible additional pathogenic role in this subgroup of patients.
Another reason to stimulate the osmolyte pathway could be based upon their known ’chaperoning‘ effects, mediating refolding of unstable or aggregated proteins,52 which makes them promising therapeutics for human protein conformational disorders. This offers perspectives for intervention in IBM, a subgroup of inflammatory myopathy currently still lacking effective treatment options. In IBM, misfolded proteins form multiple protein aggregates likely reflecting failing autophagy. The inclusions contain amyloid-β and components pointing to failing autophagic clearance, such as sequestosome 1, phosphorylated tau, and the standard autophagic marker LC3B.53 The inclusions also co-localise with the myo-inositol transporter SLC5A3.20 Whether the presence of the transporter in the aggregates represents a purely dysfunctional aspect due to trapping of the protein remains to be determined. Possibly, trapping of SLC5A3 inside the aggregates could prevent entry of the proper myo-inositol levels in the muscle fibre, disturbing the cell’s osmoregulatory system. The complex regulation displayed by osmolytes on protein folding is an open line of research. Betaine concentration-dependent effects have been reported, with betaine dosage tuning the formation/disruption balance of insoluble protein aggregates.54
An opposing therapeutic strategy should, however, also be considered, i.e. targeting the pro-inflammatory aspects of individual osmolytes if specific members of the osmolyte pathway with a pivotal role in chronic inflammation can be identified. In this respect, the expression of SLC5A3 on many muscle-infiltrating inflammatory cells20 puts this myo-inositol transporter forward as a potential target. Myo-inositol can regulate macrophage volume changes during the process of phagocytosis. The plausibility of an anti-inflammatory approach targeting osmolyte accumulators has already been shown with AKR1B1 inhibitors.55 The latter can significantly prevent inflammation build-up in the allergic lung.56 This seems an amenable approach, as the beneficiary effects of the pathway members appear to be based mostly on redundant cytoprotective activities. Reductions in individual osmolytes can be compensated for, when individual partners are being targeted, as cells can rely on a complex scala of compatible osmolytes. In muscle cells exposed to hypertonic conditions, protective increases of cell creatine levels have been observed.57
In summary, the osmolyte pathway represents potentially beneficial and adversary effects on myositis, and offers an interesting new avenue for therapeutic intervention. Full exploration of this therapeutic strategy will necessitate, however, further unravelling of osmolyte pathway activities in patient muscle tissues.