Abstract
Objectives: To evaluate an overview of the past, present, and future of robotic surgery. To provide insight and focus on the current status of the field of robotic systems for urological surgery with outcomes and discuss future perspectives in terms of other operative techniques and new robotic platforms.
Evidence acquisition: The authors undertook a non-systematic literature review using PubMed, Medline, and Google search. They used the search terms “robotic uro-surgery”, “laparoscopic”, “minimally invasive surgery”, “future of robotics”, “global robotic market growth”, “geographical distribution”, and “cost-effectiveness”.
Evidence synthesis: Robotic surgery has embraced and extended almost all aspects of uro-surgical fields since its introduction three decades ago. There are definite advantages of robots to the surgeons and patients. It has become new standard of care for many surgical procedures. Innovation and technological advances are continuing and new with more precise robots are emerging. The major downside is cost. Despite the high cost, robot market is growing.
Conclusion: Over the past decade, minimally invasive approaches have virtually revolutionised surgery and robotic surgery has accelerated these changes. Without doubt, robotic urologic surgery is here to stay and will expand further in all surgical disciplines. Utilisation of robotics should be coupled with a reduction in costs to healthcare systems, and improved clinical outcomes for the general population rather than a privileged few. Therefore, making this expensive technology more affordable must be part of the equation.
INTRODUCTION
Urology has long been recognised as a specialty that embraces technologic advances. From the earliest cystoscopes and resectoscopes to flexible ureteroscopes and laparoscopy, urologists have always been at the forefront in adopting and applying surgical technology. Hence, use of surgical robots to date has been dominated by urologists. Following the success of robot-assisted laparoscopic prostatectomy in the 1990s, robotic surgery has expanded into other domains of urological procedures worldwide. However, despite its acceptance and advantages, cost reductions are still needed.
This article explores the evolution of robotic surgery in urology over a timeline spanning the pre- and post-millennium periods. The authors also discuss potential future aspects in terms of technology, market expansion, cost, and geographical distribution.
EVIDENCE ACQUISITION
A non-systematic literature review was performed using PubMed/Medline and Google. The authors used the search terms “robotic”, “laparoscopic”, “minimally invasive uro-surgery”, “future of robotics”, “global robotic market growth”, “geographical distribution”, and “cost-effectiveness”.
EVIDENCE SYNTHESIS
Evolution of Robots and Robotic Surgery in the Pre-millennium Era (1950–2000)
The concept of robotic intervention in medicine is not new. The Industrial Revolution of the late 18th century became the instrumental phase in robotic advancement because it led to the development of key factors, including complex mechanics and electricity. In 1950, telepresence robotic arms were developed, which were direct precursors of today’s surgical robots. They were initially used in hazardous environments, such as the bottom of the ocean or in space. Further rapid advances occurred in the 1980s, with development of technologies such as microelectronics, computing, digital imaging, video electronics, and display technology. The vision of a remote surgery programme targeted towards battlefield triage, funded by the USA Defense Advanced Research Projects Agency (DARPA), was the basis for the development of surgical robots.
The very first use of a surgical robot was by Kwoh et al.,1 who used the Programme Universal Machine for Assembly (PUMA) 560 robotic system to perform neurosurgical biopsies. Subsequently, Davies et al.2 performed a transurethral resection of the prostate by PROBOT, which was designed by using a rotating blade to complete prostatic resection.3 Despite initial success, the PROBOT failed to achieve widespread acceptance. Later, ROBODOC was designed to improve the precision of hip replacement surgery4 and then a robotic-assisted percutaneous access to the kidney device was developed to facilitate stone surgery5,6 (Table 1).
However, none of the above systems were designed for laparoscopic procedures, which first derived from a collaboration between the National Aeronautics and Space Administration’s (NASA’s) Ames Research Center (Mountain View, California, USA) and researchers from SRI International (formerly Stanford Research Institute; Menlo Park, California, USA). To reduce the mortality and morbidity of service personnel in conflict zones, the USA military recognised the concept of linking surgeons (distance from battlefield) to patients (on the battlefield). Computer Motion, Inc., (Santa Barbara, California, USA) developed the Automated Endoscopic System for Optimal Positioning (AESOP) robotic platform, which helped surgeons position a laparoscopic camera by voice control. Further modifications resulted in the system being re-launched as the ZEUS operating system.7
Concurrently, Intuitive Surgical, Inc., (Sunnyvale, California, USA) released the Green Telepresence system, which became the early version of the current da Vincisystem.
The two rival systems, ZEUS and da Vinci, pushed the frontiers of minimally invasive surgery. The da Vinci platform was first used on a human patient for a cholecystectomy, which was conducted by Jacques Himpens in 1997.8
The first laparoscopic radical prostatectomy with a remote-controlled robot (da Vinci) was performed by Abbou et al.9 in 2000. The ZEUS and da Vincisystem finally unified when Computer Motion, Inc., and Intuitive Surgical, Inc., merged in 2003. As a result, Intuitive Surgical, Inc., became the sole proprietor. Since then, the da Vinci system has dominated the world of robotic surgery for almost two decades. Newer technologies with improved versions of robots have emerged and are rapidly evolving the field (Table 1).
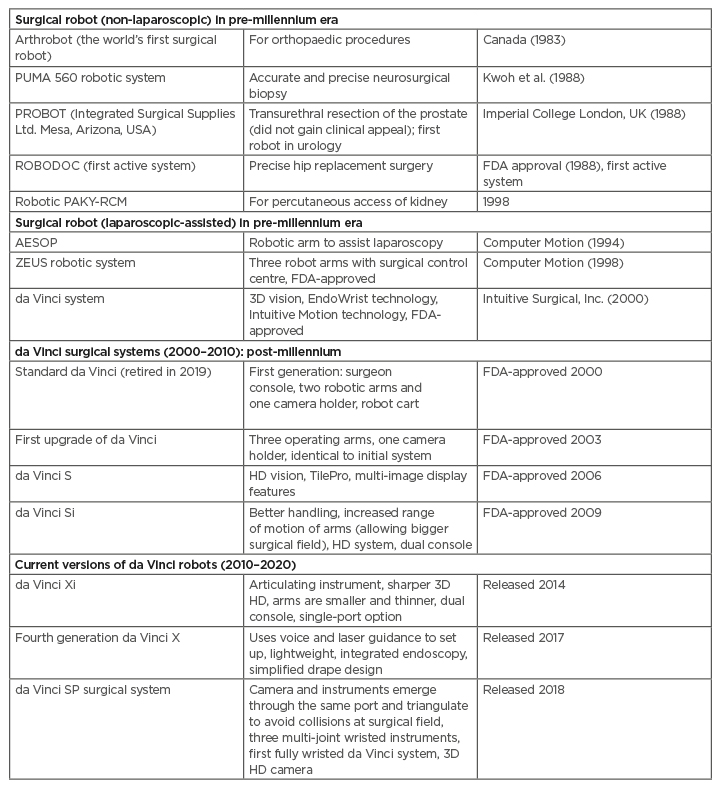
Table 1: Surgical robots in the pre-millennium era (non-laparoscopic and laparoscopic-assisted), post-millennium era, and current advanced versions.
AESOP: Automated Endoscopic System for Optimal Positioning; FDA: U.S. Food and Drug Administration; HD: high definition; PAKY: percutaneous access to the kidney; PUMA: Programmable Universal Machine for Assembly; RCM: remote centre of motion.
Robots in the Post-millennium Era (2001–2010) and Current Version (2010–2020)
The da Vinci platform produced various models for a decade. The first series of 50 robot-assisted radical prostatectomies was published by Menon et al.10 in 2002, using the first-generation of the robot. Subsequently, upgraded versions of the da Vinci system entered the market with improved technology (Table 1).
Over the past 30 years, more than 21,000 peer-reviewed articles have confirmed the safety, efficacy, and benefits of the da Vinci system (Intuitive Annual Report, 7 Feb 2020). Despite the high capital investment and high costs for disposables, updated versions of the da Vinci robot are introduced into the market continuously (Table 1).
The total number of robotic platforms installed as of 31st December 2019 was 5,582. In addition, robotic procedures of various types in hospitals throughout the world numbered 1,229,000 (data on file; da Vinci Annual Report, 7th Feb 2020), an annual increase of approximately 20%. Most surgical procedures performed robotically in the USA were in general surgery, followed by gynaecology and then urology. Conversely, outside the USA, most of the procedures were in urology.
Training Platform to Improve Skills and Performances
Approximately 400,000 deaths occur every year in the USA as a result of medical errors, making it the third-leading cause of death in the country.11,12 Surgical errors are common but potentially preventable.13 Adequate surgical skills and techniques have been directly related to patient outcomes.13,14
Robot-assisted surgery in urology is growing. The development of structured and validated training programmes is important in order to gain adequate skills and prevent poor surgical outcomes and medico-legal issues.
Technical and non-technical skills
The da Vinci robotic platform is complex and expensive. Therefore, users should be properly trained and certified. A recent paper calculated that 100–180 robotic operations are required to obtain better, or at least equivalent, results compared with open surgery.15 Another article quoted a learning curve of 8–150 procedures.16 However, surgeons experienced in both open radical prostatectomy and laparoscopic radical prostatectomy can make smooth and quicker transitions to robotics.17
Robotic platforms require both console training and patient side training. Patient side training involves patient positioning, establishing pneumoperitoneum, port placement, robot docking, and basic laparoscopic skills. Console training requires dry and wet lab simulations and supervised operating. To improve skills and performance, a variety of structured curriculums have been developed to shorten the learning curve.18,19
Non-technical skills (e.g., teamwork, leadership, and decision-making skills) are an integral part of surgical training and should be developed in parallel with the development of technical skills. One study showed that 86% of adverse surgical events were due to system errors rather than technical skills.20 Furthermore, 40% of intraoperative errors were related to failures in communication.20
Intuitive Surgical, Inc., provide integrated training with virtual simulation, data-driven optimisation, and customised solutions. Over the last 20 years, a robust training programme has therefore been built-up. This time-tested, comprehensive training programme can help develop the skills essential to using da Vinci technology.
A pathway to gain technical and clinical skills: two steps and four phases
Initially, optimisation of proficiency by developing knowledge and skills to use da Vinci technology. Second, progress with a surgeon-led series of procedures focused on clinical application, advanced techniques, and procedure refinement.
Firstly, training is centred on the core system and advanced technology. This consists of two phases: introduction to da Vinci technology (familiarisation with the system through ‘test drives’, videos, and live case observations) and da Vinci technology training (the development of technical skills via online learning, simulation, and hands-on training).
The second step is focused on progressive peer-to-peer clinical skill advancement and again consists of two phases: an initial case series plan (the integration of da Vinci technology into practice with support from experienced proctors) and continuing development (the expansion of skills through mentoring, surgeon-led programmes, and simulation).
However, a variety of new robotic systems from numerous countries are coming onto the stage. Several console-based robots for laparoscopic multi- and single-port surgery with various modifications of robotic arms, instruments, haptic feedback, eye tracking, and video technology will soon emerge. These developments with new robotic platforms would require precise, operation-specific teaching and training that could potentially be different from the current da Vinci training curriculum. In the future, the range of simulation platforms is likely to increase, with a variety of robotic training programmes and robotic simulated tasks.
Types and Levels of Robots with New Platforms
There are three main types of robotic systems currently in use in surgery: active, semi-active, and master–slave systems (Table 2).
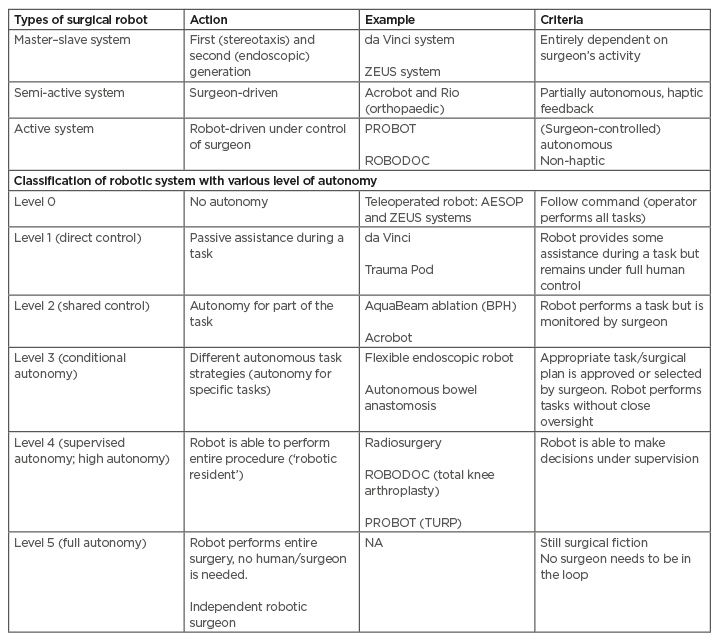
Table 2: Types and different levels of robotic systems.
Acrobot: Active Constraint Robot; AESOP: Automated Endoscopic System for Optimal Positioning; BPH: benign prostate hyperplasia; RIO: Robotic-Arm Interactive Orthopedic System; TURP: transurethral resection of the prostate.
Active systems essentially work autonomously (while remaining under the control of the operative surgeon) and undertake pre-programed tasks. The PROBOT and ROBODOC platforms are two examples.
Semi-active systems allow for a surgeon-driven element to complement the pre-programmed element of the robot system.
Master–slave systems (the da Vinci and ZEUS platforms) do not have any pre-programmed or autonomous elements.
Currently, available robots are completely dependent on human control. Attempts are being made to program the robot to operate, at least for parts of the procedure, independently (Table 2).
In future, robots may be equipped with artificial intelligence to independently perform procedures, or parts of it, by autonomous robot-assisted surgery to overcome operator-dependent variations and improve patient care. However, use of autonomous technology will come at the cost of sacrificing meaningful human control and at the expense of uro-surgical jobs. Robot-assisted surgery systems with various levels of autonomy have already been developed (Table 2).
Evidence Supporting Robot-Assisted Surgery in Urological Procedures
The majority of robotic procedures are radical prostatectomy, radical cystectomy, partial nephrectomy, and pyeloplasty. Below, the authors explore the implementation of robot-assisted surgery.
Robot-assisted radical prostatectomy
The first robot-assisted radical prostatectomy (RARP) was performed in 2000. The first series of 50 RARPs was published in 2002.10 Subsequently, numerous articles have been published. Several meta-analyses demonstrated a significant advantage in terms of potency, continence, and blood transfusion for RARP compared with open and laparoscopic approaches.21,22
Robotic surgery has revived the perineal approach for radical prostatectomy and Kaouk et al.23 presented an overview of the recent advances based on their experience with RARP.
Robotic surgery is also implemented for the surgical treatment of benign prostatic hyperplasia24 and low-risk cancer with lower urinary tract symptoms due to prostate enlargement.25
Robot-assisted radical cystectomy
Radical cystectomy with pelvic lymphadenectomy and urinary diversion is the standard of care for treating organ-confined muscle-invasive bladder cancer and refractory non-invasive disease. Despite high morbidity and the risk of perioperative mortality, open radical cystectomy remains the gold standard.26 Minimally invasive techniques are gaining momentum and popularity because they provide similar oncological outcomes with lower morbidity. The first series of robot-assisted radical cystectomies (RARCs) was reported by Menon et al.27 in 2003. Since then, there has been a dramatic increase in utilisation of RARC, from under 1% of all cystectomies performed in the USA in 2004 to approximately 23% in 2014.28
The two most recent meta-analyses found no differences regarding oncological outcomes between RARC and open radical cystectomy, but a lower 90-day complication rate, a lower transfusion rate, a shorter time to flatus, and a greater lymph node yield in patients who underwent RARC.29,30
As part of RARC, many centres are still conducting an extracorporeal urinary diversion through an abdominal mini-incision. Complete intracorporeal urinary diversion seems to fully unlock the potential of RARC. Yet, despite already being performed in some expert centres worldwide, this approach is still considered as experimental by the European Association of Urology (EAU).31 Brassetti et al.32 reported one of the largest series with long-term data about RARC with totally intracorporeal urinary diversion.
Robot-assisted radical nephrectomy
Laparoscopic radical nephrectomy is the gold standard and recommended by the EAU for patients with ≥T2 renal tumours.33 No significant differences in post-operative complications have been observed between robotic-assisted radical nephrectomy (RARN) and laparoscopic radical nephrectomy;34 however, robotic surgery is associated with a prolonged operating time and higher hospital costs. Despite this, the use of robot-associated nephrectomy in the USA increased from 1.5% in 2003 to 27.0% in 2015.34
Over the last decade, robots have been used to operate on patients with advanced renal cell carcinoma with inferior vena cava thrombus. RARN with inferior vena cava thrombectomy is a complex procedure that requires a multimodal approach involving urological, cardiothoracic, and hepatobiliary surgeons. Patients are traditionally treated with open surgery with large abdominal or thoraco-abdominal incisions. RARN with inferior vena cava thrombectomy was first reported by Abaza et al.35 Recently, Gill et al.36 presented a series of 16 patients with Level II and III inferior vena cava thrombus with good outcomes.
Robot-assisted partial nephrectomy
Robot-assisted partial nephrectomy is considered the gold standard for most small renal tumours. A meta-analysis of 4,919 patients from 25 studies showed decreased complications, less conversions to open surgery, reduced positive surgical margin rates, and a shorter warm ischaemia time in the robotic group relative to their laparoscopic counterparts.37 Complex tumours of >4 cm with high nephrometry scores are increasingly being managed using the robotic approach, with good oncological outcomes.38 Robot-assisted partial nephrectomy has proven feasible and superior to conventional laparoscopy even in solitary kidneys, multifocal renal masses, and for new or recurrent tumours in a kidney previously treated with partial nephrectomy.39
Robot-assisted nephroureterectomy
Nephroureterectomy is almost the ideal procedure to demonstrate the advantages of minimally invasive surgery in terms of cosmetics and post-operative use of analgesics.
A recently published review, despite showing some perioperative advantages for minimally invasive access, found no statistically significant differences between the laparoscopic and the robotic approach, and consequently called for larger, better designed randomised controlled trials.40
Veccia et al.41 reported a systematic review and meta-analysis of comparative outcomes of robotic versus other established nephroureterectomy techniques.
Robot-assisted retroperitoneal lymphadenectomy
Open retroperitoneal lymphadenectomy (RPLND) is still the gold standard based on oncologic outcomes and long-term follow-up data. However, complications and morbidity from open RPLND incision affect 3.7–10.0% of patients. Therefore, the demand for minimally invasive surgery for cosmetic reasons is increasing. The first case of laparoscopic RPLND was described in 1992 and that of robotic RPLND in 2006. Thirteen studies subsequently suggested that functional outcomes and in-field recurrence rates may be comparable to open approaches.42 A large case series of 47 patients with low-risk non-seminomatous germ cell tumours showed a 9% perioperative complication rate, preservation of ejaculation in 100% of patients, and a recurrence-free rate of 97% at 16 months.43 Another study showed no retroperitoneal recurrences at 22 months and a minor complication rate (Clavien–Dindo Grade II) of 17%.44 Rocco et al.45 reported the largest series of primary robot-assisted RPLND for non-seminomatous germ cell tumours.
Robot-assisted pyeloplasty
The published data regarding robot-assisted pyeloplasty show excellent results and success rates (of 94–100%), as well as very good results in redo operations after failed primary procedures (with success rates of 78–94%). A meta-analysis of nine published studies on 277 robotic cases and 196 laparoscopic cases showed no differences between the two techniques apart from a shorter operative time for the robotic operation.46
Robot-assisted laparoscopic ureteric re-implantation
Over the past decade, there has been an increasing shift toward robot-assisted laparoscopic distal ureteric reimplantation. The first reported robot-assisted laparoscopic ureteric re-implantation was performed by Yohannes et al.47 in 2003. A recent review by Asghar et al.48 in 2020 showed advantages in terms of blood loss, length of hospital stays, and post-operative pain for the robotic compared with the open approach.
Under scrutiny: recent expansion of other robotic uro-surgery procedures
Robotic surgery is being explored for adrenal surgery. Heger et al.49 recently published a meta-analysis of 1,710 patients who underwent either a minimally invasive (robotic or laparoscopic) or an open adrenalectomy. Blood loss was lowest in the robotic group. A significant reduction in the length of stay compared with conventional laparoscopy was also recorded.Ye et al.50 described a novel robotic adrenal enucleation technique.
Two randomised trials comparing laparoscopic and robot-assisted sacrocolpopexy showed higher costs, a longer operative time, and more post-operative pain in the robotic group.51
Robotic platforms have allowed lymph node dissection for several genitourinary cancers.52 In children, the success rate of robotic-assisted surgery for the treatment of vesicoureteral reflux was below 90% whereas the success rate of open extravesical ureteric re-implantation was approximately 98%.53
The Future of Robotic Surgery
Despite the high cost of robots, there is a continuing expansion of robotic surgical procedures. Although adoption of robots for surgery was introduced in the 1990s, only 15.1% of all common operations in the USA are performed robotically (increased from 1.8% in 2012 to 15.0% in 2018).54 Robotic-assisted laparoscopic prostatectomy increased from 0.7% in 2003 to 42.0% in 201055 and by 2014, RARP accounted for up to 90% across the USA.56 However, this figure is likely to be much lower outside America.
The global market for surgical robots keeps growing. The initial capital investment for a da Vinci system is 1.2–1.5 million USD, with an annual maintenance cost of 100,000 USD and an average disposable instrument cost of 1,400–1,700 USD per case. Bolenz et al.57 compared costs for a RARP with laparoscopic and open procedures. They calculated the costs of RARP, laparoscopic RP, and open RP as 9,450 USD, 5,687 USD, and 4,437 USD per operation, respectively.
As there are no competitors, da Vinci do not yet have to compromise on the cost because of their monopoly. A centre needs to perform 100–150 cases of robot-assisted surgeries per year to be cost-effective.58 The fastest growing Asian and other developers are coming to the market with low-cost surgical robots that will soon challenge the expensive da Vinci monopoly while also providing the same or better surgical outcomes (Table 3).
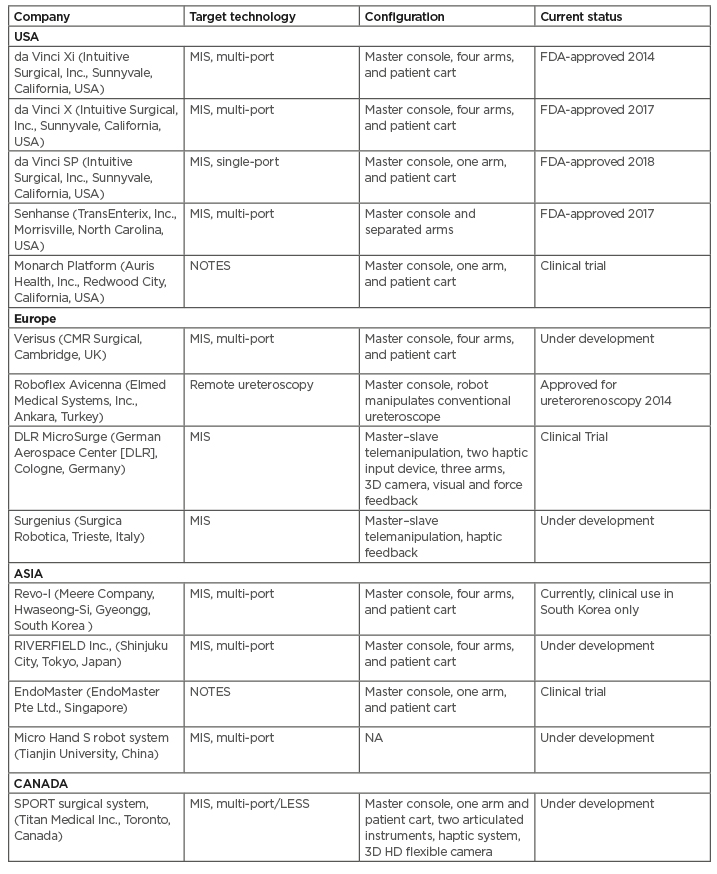
Table 3: Recent and future emerging robotics in urology.
FDA: U.S. Food and Drug Administration; LESS: laparoendoscopic single-port surgery; MIS: minimally invasive surgery; NA: not applicable; NOTES: natural orifice transluminal endoscopic surgery; SPORT: Single Port Orifice Robotic Technology.
Western countries have possibly already reached, or will soon reach, the peak and plateau level for the installation of new robots. The Asia-Pacific and South American markets are the target of future robot installations and expected to grow very fast. Currently, differences in surgical robot density are marked.
In Brazil, there is one robot per 5.1 million people, in Europe there is one robot per 998,000 people, and in the USA there is one robot per 112,000 people.59 Moreover, in emerging countries, most robotic platforms are usually concentrated in affluent cities and at private healthcare institutions. Only affluent patients pay out of their pocket because insurance, if it even exists, does not cover the cost of robotic operations .
However, the ongoing COVID-19 crisis will likely be followed by a global recession in the near future. The costs of robotic surgery have become the prominent factor driving treatment and investment decisions. The COVID-19 pandemic led to a 40% reduction in its shares on the stock market in late March 2020. Globally, procedures performed using the da Vinci surgical system decreased by 19% worldwide in Quarter 2 of 2020 compared with 2019 because elective procedures were either cancelled or postponed. Quarter 3 revenue was 1.1 billion USD, down 4% from last year. During Quarter 3, Intuitive Surgical, Inc., shipped 195 new da Vinci surgical systems, a 29% decline from the same quarter in 2019. The global robotic surgery market is expected to decline from 5.04 billion USD in 2019 to 4.84 billion USD in 2020, which is a decrease of 3.91% from 2019, as a consequence of the COVID-19 crisis. The market will eventually stabilise and is expected to reach 7.71 billion USD in 2023.60
A future ideal robotic system should be cost-effective, easy to use, easy to set up, and portable so that it can be used in different locations and settings.
The main limitation of this article is that it is a non-systematic literature review; therefore, there is a possibility of subjective bias.
CONCLUSION
Over the past decade, minimally invasive approaches have revolutionised surgery, and robotic surgery has accelerated these changes. Without doubt, robotic urologic surgery is here to stay and will expand further in all surgical disciplines. Utilisation of robotics should be coupled with a reduction in costs to healthcare systems and improved clinical outcomes for the general population rather than a privileged few. Therefore, making this expensive technology more affordable must be part of the equation.