Abstract
Prostate cancer (PCa) is the second most frequently diagnosed cancer in males; early-stage PCa is asymptomatic, and PCa has an indolent course. The current standard of care of systematic transrectal biopsy (SBx) is preferred by urologists because of its ease of use and portability, despite its shortcomings in cancer detection rate. The advent of multi-parametric MRI (mpMRI)-enabled PIRADS protocol for lesion diagnosis and characterisation has helped minimise unnecessary biopsies, supporting the active surveillance protocol of patients with low-risk PCa. The use of annotated pre-procedure MRI fused with real-time ultrasound (US) to guide biopsies has been gaining traction in clinical use, but the challenges in registration of two different modalities, gland deformation due to the probe, and significant learning curve associated with fusion have resulted in slower than expected adoption in routine clinical practice. Moreover, the fusion biopsy has only marginally improved cancer detection rate, with a complex workflow. Higher infection rate with transrectal prostate interventions has resulted in an increased use of the transperineal approach to guide biopsies and therapies. There has been significant progress made in the development of point-of-care, portable MRI systems for specific use. In this report, the authors discuss the recent developments in office-based prostate interventions that have occurred with the arrival of low-field MRI systems. The smaller footprint of the low-field system avoids the high costs associated with the installation and management of regular MRI. Additionally, the availability of transperineal MRI visible grid makes the targeting and guidance processes relatively easier with a less-steep learning curve. Since the system uses pre-plan high-field MRI acquired in the same transverse orientation as the low-field MRI, registration errors are smaller than the MRI–US registration. The use of MRI to target lesions has reduced the number of cores sampled, benefiting the patient with early clinical study showing significantly higher cancer detection rate than SBx.
INTRODUCTION
Prostate cancer (PCa) is the second most prevalent cancer among males in the USA, with >180,000 new cases diagnosed in 2016.1 The 5-year survival rate for patients initially diagnosed with local or regional prostate cancer is almost 100% but falls to 31% for those with an initial diagnosis of PCa that has metastasised.1
The current diagnostic pathway of PCa requires males with elevated prostate-specific antigen and/or an abnormal digital rectal examination to undergo a transrectal ultrasound (TRUS)-guided biopsy.2-6 As a blind systematic biopsy (SBx), which randomly samples prostate tissue, it can lead to missing or under-diagnosing clinically significant cancer and over-diagnosing clinically insignificant disease.4-5,7 Moreover, TRUS biopsy is associated with morbidity and can also sometimes cause life-threatening sepsis.2,7
An alternative to the transrectal approach is the transperineal approach (TPUS), which allows for easier access to all parts of the prostate and avoids contamination by the rectum, therefore resulting in greater accuracy and lower infection rates.8 Recently, the European Association of Urology (EAU) published a position paper9 recommending TPUS as a first choice due to its least-contaminating approach, reducing the rate of infection complications.
In recent years, multi-parametric MRI (mpMRI) has been reported as an efficient tool to guide prostate biopsy decision-making.2,10 Indeed, EAU guidelines recommend performing mpMRI before prostate biopsy in biopsy-naïve patients, as well as patients with prior negative biopsy with a clinical suspicion PCa.11 Moreover, techniques such as MRI–ultrasound (US) fusion biopsy have emerged as a way to target lesions more precisely, compared to the traditional systematic transrectal and transperineal US biopsy techniques. These targeted MRI-based approaches have been reported to show improvement in rates of cancer detection compared to a SBx12-14 but do have shortcomings.
Most common MRI systems, 1.5 Tesla (T) or 3T scanners, are very expensive to purchase and maintain, as well as time consuming and uncomfortable for patients15,16 for image-guided interventions. This has led to the development of portable, less expensive, and office-based low-field MRI systems.17,18
In this review, the authors describe the recent developments of a U.S. Food and Drug Administration (FDA)-cleared, first of its kind, single-sided, low-field, open, point-of-care MRI system and its role in office-based prostate interventions.
CURRENT DIAGNOSTIC PATHWAY
Systematic Transrectal Biopsy
TRUS SBx is the most common approach for the initial diagnosis and grading of prostate cancer. It consists of 10–12 core biopsies,19 and is usually performed in the urologist’s office under local anaesthesia. It is easy to perform, widely available, and well tolerated by patients.20 SBx provides a non-targeted, blinded sampling of the prostate (usually the periphery of the prostate), and is associated with missing or under-diagnosing clinically significant cancer and over-diagnosing clinically insignificant disease.2 One consequence is over-treatment of patients with clinically insignificant disease, which even if left untreated, have little to no clinical impact on an individual’s remaining life.21 Moreover, over-treatment often leads to side effects like erectile dysfunction and urinary incontinence.21
Another consequence is incorrect risk stratification due to under-sampling or false negative biopsies. It occurs in up to 30% of cases in some series,22 with the PROMIS trial reporting 52% false negative rates,2 which leads to under-grading and missing clinically significant disease on initial biopsy. Interestingly, increasing the number of cores has a marginal increase in overall detection rate, while it appears to increase the rate of insignificant PCa detection.23
Furthermore, SBx is associated with morbidity, mainly in the form of haematuria, haematospermia, pain, and urinary retention.2,7 In addition, as long as the biopsy needle passes through the rectum, it can lead to urinary infection and even life-threatening sepsis. Higher rates of infection from TRUS has led to the use of TPUS biopsy, but the increased burden of resource utilisation combined with the lack of Level-1 evidence has limited wider adoption.24
MRI–Ultrasound Fusion Biopsy
In recent years, mpMRI appears to be a complementary screening and diagnosis tool in the PCa diagnostic pathway. Its ability to detect PCa has been extensively validated in several previous studies.6,25,26 A recent meta-analysis looking at the ability of mpMRI to detect PCa showed that mpMRI had high specificity 0.88 (95% confidence interval: 0.82–0.92) and sensitivity of 0.74 (95% confidence interval: 0.66–0.81), with negative predictive value having broader range of 0.65–0.94.27
Moreover, MRI-targeted biopsy (MRI-TB) has emerged as a way to more precisely target lesions under ultrasound guidance seen on a prior mpMRI scan. These targeted MRI-based approaches have been reported to show improvement in rates of cancer detection, decrease the number of unnecessary biopsies, and reduce the treatment of clinically insignificant disease.12-14,26,28
Three clinical trials have demonstrated this approach in biopsy-naϊve males. In the MRI-FIRST trial, Rouviere et al.10 conducted a prospective, multi-centre study comparing MRI-TB to SBx within the same patient. They found that detection of clinically significant PCa was not different in one of the two techniques (32% versus 30%; p=0.38), but the detection was improved by combining both techniques. The 4M trial29 was also a prospective, multi-centre study comparing MRI-TB to SBx within the same patient. The results showed a similar detection rate of clinically significant PCa (25% versus 23%; p=0.17). However, MRI-TB detected a lower proportion of clinically insignificant disease (14% versus 25%; p<0.001). Lastly, the PRECISON trial30 was a multi-centre, randomised, non-inferiority trial where patients were randomised to either traditional systematic TRUS-biopsy or MRI-TB. Unlike the other trials, MRI-TB detected more clinically significant PCa than SBx (38% versus 26%; p=0.005). Furthermore, MRI-TB detected less clinically insignificant PCa (9% versus 22%; p<0.001). These findings have been confirmed by systematic reviews,31-34 both in biopsy-naïve patients and those with prior negative biopsy, and in the active surveillance population.
Limitations of Fusion Biopsy
Despite all the advantages of MRI-TB, the rate of adoption in routine clinical practice has been slower due to certain issues. The MR image used to guide biopsies are a composite of mpMRI comprising T2-weighted imaging (T2W), diffusion-weighted, and dynamic contrast-enhanced images.35 Though there are automated tools available to create the composite image, it still adds overhead to the workflow. Registration of mpMRI to US poses problems since there exists different techniques of registration such as cognitive registration, MRI-US fusion registration, and in-bore MRI-targeted registration,36 resulting in errors in lesion targeting and resulting in significant impact on clinical outcomes. The median target registration error between MRI and 3D US is 3.8–5.6 mm, and 2D US is 2.5–3.6 mm, dependent on operator experience.37 In different trials, none have shown a superiority in detecting clinically significant PCa, but the detection rate was higher when MRI-TB and SBx approaches were combined.38-40
There has been evidence that MRI’s ability to identify PCa varies with factors like reader expertise, grade and disease volume, MRI sequences, etc.27,28 Despite the existence of Prostate Imaging Reporting and Data System (PI-RADS) guidelines that offer a standard to radiologists for reporting prostate MRI,41 a multi-centre study including six prostate radiologists found a moderate reproducibility and a high inter-centre and -reader variation of mpMRI.42 Moreover, in a Cochrane systematic review,43 a negative mpMRI falsely predicted the absence of clinically significant prostate cancer in 9% of males.
As there is a learning curve for the interpretation of prostate mpMRI, there is also one for all techniques of MRI-TB. A retrospective study of a prospective cohort of 1,813 males with prostate biopsy demonstrated a 26% increase in clinically significant PCa detection rate in a 4-year period.44
In spite of the benefits, in-bore MRI interventions are limited as they are time-consuming, create higher demand on resources, and require MR safe instrumentation. Additionally, the economics associated in the purchase and maintenance of MRI systems are prohibitive for specialised use such as image-guided biopsies and require strong-magnetic-field-specific facility upgrades for housing the system. Highly specialised expertise, increase in MR safety training, and hazardous material protocols needed for appropriate cryogen use are other significant drawbacks. The size of the MR bore imposes restrictions on patient selection in addition to the inability to accommodate claustrophobic patients. Moreover, the demand for routine diagnostic use prohibits the use of in-bore interventions except for select academic and research centres.
LOW-FIELD MRI SYSTEM
Low-Field MRI-Guided Biopsy
Recently, with the development of better magnet design, radiofrequency, and gradient technologies, there is an increased interest towards low-field MRI for targeted applications. The very first studies in this field were published in the early 80s and 90s, in particular for neurology imaging and interventional applications.45-47
The Promaxo MRI System (Promaxo Inc., Oakland, California, USA) is the first of its kind: a single-sided, office-based, low-field MRI system with an open design to accommodate patients of all sizes and types, including those with claustrophobia. It is a cleared by the U.S. FDA and is intended to be used for targeting prostate lesions under MR guidance in alignment with the current standard of care. Having a smaller footprint, with a field strength of 65 mT and pre-programmed pulse sequences for optimal workflow, requiring neither significant facility upgrades nor operating costs, makes it suitable for office-based biopsies. A smaller footprint (Figure 1), with low energy utilisation and the absence of any hazardous materials such as cryogens, makes it ideal for office-based procedures without requiring any significant facility upgrades.
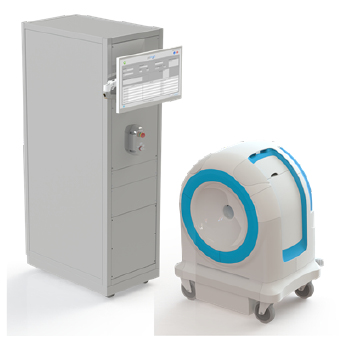
Figure 1: Promaxo MRI system.
The system comprises two main components: the electronic panel (left) and the magnet cart (right). A patient wearable receive coil is not shown in the image.
MRI-Targeted Biopsy Clinical Workflow
The clinical workflow consists of regions of interest identified and annotated on commercial whole-body 3T T2W images obtained in advance by a board-certified radiologist using the Promaxo DICOM Viewer. The annotated images are uploaded to the Promaxo MRI System prior to the procedure date. For the MRI-TB procedure, patients are placed in a high lithotomy position (Figure 2) with their perineum close to the centre of the single-sided MRI field of view (120 mm) magnet, such that the entire prostate gland would be visible in the resulting scan. A 5-channel surface coil containing an MR-visible biopsy grid (Figure 1) for transperineal access encloses the pelvic region, and an additional 1-channel fiducial and back coils are placed on the pelvis and lower back region, respectively.
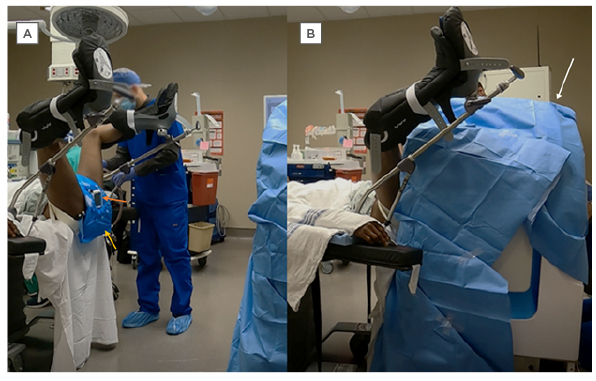
Figure 2: A subject in a lithotomy position for a pelvic scan.
A) The yellow arrow points to the surface coil and the orange arrow points to the MRI visible biopsy grid. B) A lateral view of the patient positioned with the pelvis against the Promaxo MR scanner (behind the surgical drape) for the procedure.
The Promaxo MRI protocol starts with a 4-minute localiser scan to locate the prostate (repetition time/echo time: 1,200/45.93 ms; sequence [spin echo]: RARE; image type: cartesian; percent sampling: 50%; echo train length: 8). Once positioning is confirmed, a T2W MRI scan (repetition time/echo time: 1,500/15.3 ms; radial sampling; echo train length: 7) is acquired. Forty slices of T2W images in the axial plane are obtained with a 120×120 mm field-of-view, and are reconstructed to a field-of-view of 180×180 mm, with 2.8 mm slice thickness, resulting in an approximate voxel size of 9 cc. On the day of the procedure, patients undergo sedation as preferred by the practice.
A board-certified urologist uses the Promaxo graphical user interface to manually co-register the annotated 3T T2W images with the acquired Promaxo MRI T2W scans (Figure 3), and identifies the lesion targets based on the physical template co-ordinates and depth displayed on the registered images. Following registration and target planning, the needle(s) are inserted transperineally through the designated co-ordinate location and tissue samples are extracted. The grid is designed to accommodate an MR-safe 20 cm biopsy gun with an 18 G biopsy needle and either a 14 G or 17 G cannula.
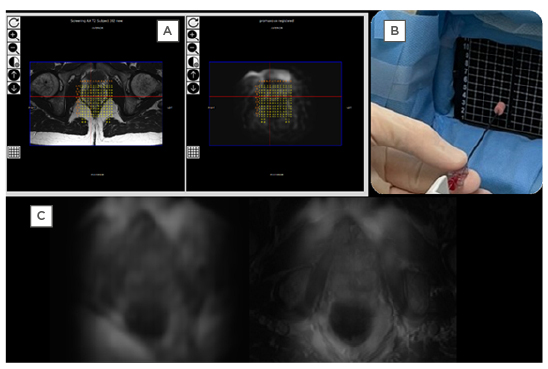
Figure 3: Example of the Promaxo in use with prostate images.
A) 3T T2W images on the left (with the annotation) is co-registered with T2W Promaxo MR image on the right (shown here in Promaxo viewer). The red circle shows the target, once identified by the urologist. B) The appropriate needle is inserted, and samples are taken as shown. C) Left: axial T2W slice; right: T2W axial Promaxo MR fused with high-field (1.5T) MR.
3T: 3 Tesla; MR: magnetic resonance; T2W: T2-weighted.
Clinical Experience
The system currently is in use at Mississippi Urology, a community-based practice in Jackson, Mississippi, USA. Using an institutional review board-approved research protocol (WIRB #20203968), 16 males (average age: 67±8 years) referred for SBx to a community urology practice were consented for MRI-TB with the Promaxo MRI for biopsy guidance. Cancer was found in six of 16 patients by both SBx and MRI-TB, while MRI-TB found cancers in six more patients resulting in a 37% higher cancer detection rate with MRI-TB. Initial results from this ongoing study demonstrate in-office prostate MRI-TB biopsy with a low-field MRI system is feasible, and confirm the benefits of co-registering high-field with low-field MRI for improved guidance to target lesions.
Future Possibilities
The use of artificial intelligence (AI) in healthcare is ubiquitous from automated segmentation of mpMRI,48 de-noising of medical images,49 and treatment.50 AI technology enables health professionals to deliver better care for their patients and increase productivity, while improving patients’ experiences and outcomes. Deep-learning-based U-Net architecture51 is used in Promaxo MR to reduce noise from the system and remove artefacts due to the inhomogeneous B0 field, non-linear gradients, under-sampling of k-space, and image reconstruction to enhance low-field MR.
The growing interest for the case of simpler and cheaper prostate MRI,52 followed by the recent recommendation by the UK’s National Institute for Health and Care Excellence (NICE),53 for the use of mpMRI, the authors predict low-field open systems such as Promaxo MRI will play a significant role. As an office-based system, board-certified urologists would be able to readily screen all males >50 years old with pre-programmed prostate-specific sequences and the help of AI to enhance signal and contrast to noise along with tissue characterisation.
The open design of the MRI and the ability to use it in an outpatient centre or ambulatory surgical centres enables a suitable environment for targeted robotic procedures with greater precision. Moreover, this platform could be used as a treatment tool under live MRI such as focal therapy, radiation treatment, and surgery.
CONCLUSION
The limitations imposed by high-field MRI systems for prostate interventions in an outpatient centre or ambulatory surgical centre have enabled the development of better magnet design, radiofrequency, and gradient technologies for low-field MRI. The Promaxo MRI System is the first single-sided, low-field, and open MRI system developed and cleared by the U.S. FDA for prostate biopsies of target lesions under MRI guidance. The smaller footprint of the system is suitable for office-based procedures and allows board-certified urologists to perform prostate interventions in outpatient settings. With relatively straightforward navigation and registration techniques, Promaxo MRI reduces registration errors and improves target rates for therapies. The authors believe that it will improve the rate of clinically significant cancer detection and results for targeting therapies of PCa. This review provides important advances of the low-field MRI system for prostate interventions and its future possibilities in term of interventional applications.