Abstract
Second-generation drug-eluting stents are currently considered the standard of care in patients undergoing treatment for coronary artery disease with percutaneous coronary intervention. Despite significant improvements in stenting technology and stent material over the past three decades, the concern that a permanent metallic prosthesis within the coronary vasculature can serve as a trigger for stent-related adverse events, mainly stent thrombosis and in-stent restenosis, still persists. In order to overcome the disadvantages of drug-eluting stents there has been a robust development in the field of bioresorbable coronary scaffolds (BRS). These devices aim to provide temporary scaffolding to restore vessel patency and, after serving its purpose, fully degrade and thus allow restoration of vasomotion along with luminal enlargement. The initial experience with bioresorbable scaffolds in low-risk patients presenting with simple lesions was satisfying and generated optimism among interventional cardiologists by promising better patient outcomes. However, the unrestricted use of these devices in patients presenting with a higher baseline risk and more complex lesions came at the cost of alarmingly high rates of adverse cardiac events, especially the late device thrombosis. Although its non-inferiority compared to metallic everolimus-eluting stents was formally met in the clinical trials, there was a clear trend towards an increased occurrence of myocardial infarction and device thrombosis during the first year after device implantation, which persisted even at long-term follow-up raising concern on the future of BRS. This review article discusses the development, design, clinical data, and future directions in the field of BRS.
INTRODUCTION
Techniques for coronary artery revascularisation using percutaneous coronary intervention (PCI) have undergone significant advancements over the past few decades. The first major advancement in the field of PCI involved the use of balloon angioplasty; however, it was associated with a restenosis rate of about 40% and an unfavourable coronary anatomy precluded balloon angioplasty in 50% of cases.1 Coronary stents were created with the advent of bare-metal stents (BMS) to overcome these shortcomings and marked the second phase of revolution in PCI. BMS resolved the issue of acute vessel closure by sealing the dissection flap and preventing elastic recoil, as was shown in the landmark BENESTENT trial, with the rate of subacute occlusion reduced to 1.5% and restenosis rates reduced from 32% to 22% at 7-month follow-up.2 However, the use of BMS was associated with increased incidence of neointimal hyperplasia and in-stent restenosis, which led to repeat revascularisations and hence limited its widespread adoption.3 In order to overcome the limitations of BMS, drug-eluting stents (DES) were developed, incorporating controlled local release of anti-proliferative agents with the aim of preventing neointimal hyperplasia and reducing the risk of restenosis. The introduction of DES heralded the third phase of revolution in the field of PCI and demonstrated a dramatic reduction in rate of restenosis compared to BMS.4 The frequency of stent thrombosis with second-generation DES are now reported to be at an all-time low at <1%.5 Despite these improvements, newer-generation DES have not managed to address all the limitations of permanent coronary stents, such as the persistent risks of target lesion revascularisation and neoatherosclerosis, hindrance of late lumen enlargement, and the lack of reactive vasomotion in the stented vessel. Furthermore, implanting DES can hinder surgical revascularisation, jail side branches, require long-term antiplatelet therapy, create blooming artefact on imaging, and predispose the vessel to late stent thrombosis.6
Everolimus-eluting bioresorbable coronary scaffolds (BRS) were presented as the ‘magic bullet’ for overcoming the limitations and adverse effects associated with permanent indwelling drug-eluting metallic stents. However, mid-term and long-term data on the leading BRS (Absorb, Abbott Vascular, Santa Clara, California, USA) contradicted the expected advantages of the BRS and showed a higher rate of late scaffold thrombosis.7-9 This article will briefly review the key concepts in and current clinical evidence for BRS.
CURRENTLY AVAILABLE BIORESORBABLE CORONARY SCAFFOLD TYPES AND THE CLINICAL EVIDENCE
A summary of currently approved and under investigation bioresorbable scaffolds with their technical specifications is presented in Table 1.
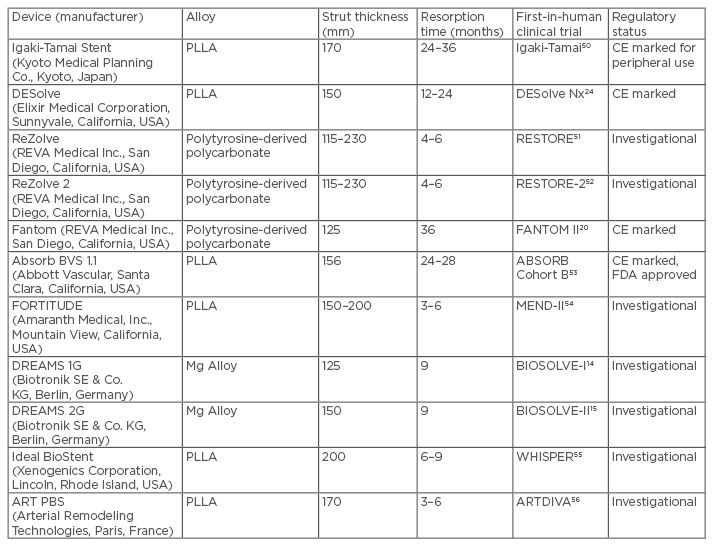
Table 1: Summary of currently approved and under investigation bioresorbable scaffolds with their technical specifications.
CE: Conformité Européene; FDA: U.S. Food and Drug Administration; PLLA: polylactic acid.
Metal Alloy Scaffolds
So far, only two metal alloy-based BRS have been developed, which are composed of either iron or magnesium.10 The absorbable metal stent (AMS)-1 (Biotronik, Berlin, Germany) (Figure 1) was the first metallic scaffold created and was composed of a non-drug-coated magnesium alloy with a strut thickness of 165 µm and resorption time of 4 months. The AMS-1 was evaluated in a prospective, multicentre, non-randomised clinical trial (PROGRESS AMS) for neointimal formation and vascular remodelling. No safety concerns, such as myocardial infarction, stent thrombosis, or death, were observed; however, the restenosis rate was unacceptably high (47.5% at 4 months and 45% at 1 year), requiring repeat revascularisation.11 Patients enrolled in the trial had intravascular ultrasound (IVUS) at 4 months, which revealed late lumen loss due to early recoil resulting from the scaffold dissolving at a much faster rate than expected.12 This prompted the development of second-generation AMS-2 and AMS-3 (Figure 1) with the purpose of extending the duration of time needed for complete degradation.13 AMS-3 DREAMS was tested in a prospective, non-randomised, multicentre trial study BIOSOLVE-I. At 12 months, target lesion failure was observed in 3 (7%) of the 43 patients.14 DREAM has since been modified to the DREAMS-2 or Magmaris scaffold, which incorporates radiopaque markers at both ends of the device to improve placement and elutes sirolimus instead of paclitaxel. The safety and performance of this device were examined in a prospective, multicentre, non-randomised trial study BIOSOLVE-II and demonstrated a significantly improved in-segment late lumen loss (0.27 mm) and in-scaffold late lumen loss (0.44 mm) at 6 months.15
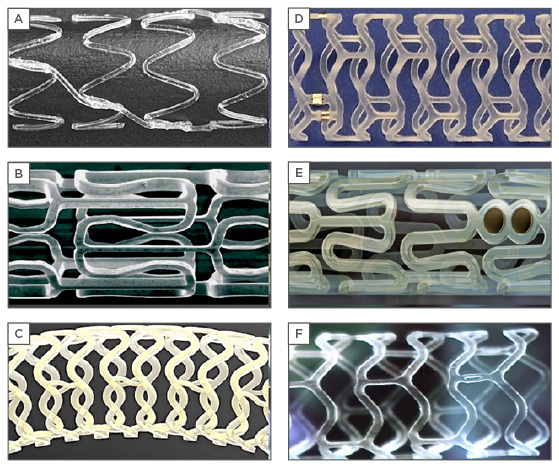
Figure 1: Design of various bioresorbable coronary scaffolds.
A) Igaki-Tamai; B) absorbable metal stent; C) Fantom; D) DESolve; E) Absorb GT1; F) Fortitude.
ReZolve Scaffold
The ReZolve scaffold (REVA Medical Inc., San Diego, California, USA) (Figure 1) consists of an absorbable tyrosine-derived polycarbonate polymer coated with sirolimus, strut thickness of 122 µm, and a resorption period ranging from 4–6 months.16 The first-generation scaffold was tested in the RESORB trial. This was a prospective, non-randomised, single-arm study enrolling 27 patients showing a higher rate of target lesion revascularisation (66.7%) at 6 months.17 After redesigning the device using a proprietary desaminotyrosine polycarbonate polymer coated with a sirolimus antiproliferative agent, the newer generation scaffold named Fantom (REVA Medical Inc.) was created. It had a strut thickness of 125 µm and a resorption time of 36 months.18,19 The safety and performance of the Fantom device are being evaluated in the FANTOM II trial. The trial is currently being expanded to determine the safety and effectiveness in more complex cases, including two lesions in one or more arteries and lesions >20 mm in length.20
DESolve Scaffold
The DESolve BRS (Elixir Medical, Sunnyvale, California, USA) (Figure 1) is composed of a poly lactic acid backbone coated with myolimus. It has a strut thickness of 150 µm and a resorption time ranging from 12 to 24 months.21 Uniquely, it also contains a self-correction feature of increasing the scaffold dimensions to minimise mal-positioning if underdeployed.22 The latest generation device DESolve-150 has a novolimus drug coating and is estimated to be completely reabsorbed within 2 years.23 The efficacy of DESolve-150 was assessed in the DESolve Nx trial, which showed late lumen loss at 6 months to be 0.20±0.32 mm and a major adverse cardiovascular events rate at 24 months of 7.4% with no definite scaffold thromboses.24
Absorb Scaffold
The Abbott Vascular everolimus bioresorbable vascular scaffold (BVS) is the most widely used and investigated BRS. The Absorb GT1 BVS (Abbott Vascular) (Figure 1) obtained a Conformité Européene mark in 2010 and was approved by the U.S. Food and Drug Administration (FDA) in July 2016 for use in the USA.25 Seven randomised clinical trials comparing Absorb BVS with everolimus-eluting metallic stents have been conducted.9,26-32 Six of these trials (ABSORB II, ABSORB III, ABSORB China, ABSORB Japan, EVERBIOII, and AIDA Trial) included patients presenting with stable ischaemic heart disease, whereas one study (TROFI II)29 included patients with ST-segment-elevation myocardial infarction. At 1-year follow-up, the results of these studies suggested that there were no differences in the rates of the composite patient orientated and device-orientated adverse events between the two devices. However, long-term data from the aforementioned trials and a recently published meta-analysis have raised safety concerns, with the Absorb BVS showing a significant increase in the risk of target lesion failure (driven by a significant increase in target vessel myocardial infarction and ischaemia-driven target lesion revascularisation) and scaffold thrombosis compared with everolimus-eluting stents.8 The possible proposed mechanisms for the excessive thrombotic event in the BVS group include strut malapposition (either persistent or late acquired); late device discontinuity, which is a programmed phenomenon in the bioresorption process of the polymeric device; delayed vessel healing; and neoatherosclerosis.33-36
ABSORB III trial was a pivotal study that paved the way for the BVS device approval in the USA.27 In this multicentre, single-blinded, active-treatment, randomised trial, 2,008 patients with stable or unstable angina due to noncomplex obstructive coronary artery disease were randomly assigned in a 2:1 ratio to receive either the Absorb BVS (n=1,322) or Xience stent (Abbott Vascular) (n=686).27 Target-lesion failure at 1 year was observed in 7.8% (99 of 1245 patients) receiving an Absorb BVS versus 6.1% (44 of 726 patients) receiving Xience.27 No significant difference was noted between BVS versus metallic stent in rates of cardiac death (0.6% and 0.1%, respectively; p=0.29), target-vessel myocardial infarction (6.0% and 4.6%, respectively; p=0.18), or ischaemia-driven target-lesion revascularisation (3.0% and 2.5%, respectively; p=0.50).27 Device thrombosis was noted in 1.5% of patients receiving the Absorb BVS versus 0.7% of patients receiving Xience within 1 year (p=0.13).27 Recently, 2-year data from ABSORB III was reported with the rate of target lesion failure being 11.0% in the Absorb group and 7.9% in the Xience group (p=0.03) along with a higher rate of device thrombosis (1.9% versus 0.8%) with BVS.37 This prompted the FDA to issue a safety notice limiting the use of Absorb BVS to centres participating in clinical registries. The 3-year outcomes from the ABSORB II trial were also reported recently and revealed a two-fold increased risk of target vessel myocardial infarction and late scaffold thrombosis when compared to the Xience device along with six reported cases of definite very late scaffold thrombosis (>365 days).9 In addition, the co-primary endpoint of superior vasomotor in Absorb BVS was not met, an unexpected finding given the rate of resorption. This was due to the fact that the metallic stents exhibited an unexpected change in diameter not previously reported in the literature.38 However, the overall vasodilation of the scaffold observed in ABSORB II study (0.047 mm [standard deviation (SD): 0.11]) was very similar to the vasodilation observed in the first-in-human study at 3 years (0.054 mm [SD: 0.12])25 and in the ABSORB Japan randomised trial at 2 years (0.06 mm [SD: 0.14]).26 Future trials might consider alternative imaging, different vasodilator responses, or later follow-up to confirm whether there is a true vasomotor advantage for the bioresorbable scaffold or whether this proposed benefit is not realised in practice when compared with contemporary metallic DES.
The most recent clinical data for Absorb BVS comes from the Amsterdam Investigator-Initiated Absorb Strategy All-Comers Trial (AIDA). It was a single-blinded, multicentre, investigator-initiated, noninferiority, randomised clinical trial that included 1,845 patients selected to receive either a BVS (n=924) or a metallic stent (n=921).31 At 24 months, target vessel failure occurred in 11.7% of patients receiving the Absorb BVS and 10.7% of patients receiving the Xience device, which was statistically nonsignificant.31 Also, there was no difference in the risk of target vessel revascularisation events or cardiac related deaths. However, a significantly elevated risk of target vessel myocardial infarction in the BVS versus metallic stent group (5.5% versus 3.2%, respectively; p=0.04) prompted an early report of the data due to safety concerns.31 Ongoing trials, such as the ABSORB IV trial, continue to determine the long-term safety and efficacy of Absorb BVS by examining rates of target lesion failure within 5 years of device placement.
PATIENT AND LESION SELECTION FOR BIORESORBABLE VASCULAR SCAFFOLD
Lessons learned from the clinical trials conducted with first-generation Absorb BVS have sounded a note of caution to the operators using BVS. We discuss a few of the important considerations that should be taken into account prior to implanting a BRS.
Large vessels with a luminal diameter >4.0 mm should be excluded from BVS implantation, since the largest available BVS diameter is 3.5 mm with a corresponding maximum post-implantation balloon diameter of 4 mm. Overexpansion of BVS could lead to fracture, whereas leaving the BVS malapposed can cause scaffold thrombosis.39,40 In contrast, BVS should not be deployed in very small vessels, especially those with a diameter <2.5 mm. This is based on the fact that BVS strut thickness is 156 µm and if implanted in a small vessel, the final lumen area after BVS implantation would become excessively narrow, thereby increasing the risk of scaffold thrombosis.
The operator should have a low threshold for intravascular imaging use to confirm lumen/vessel diameter when it is difficult to define diameter before deciding to use BVS, especially in small/ large vessels and/or diffuse lesions.41 Operators should also be careful in making a decision to implant BVS in lesions with large differences between proximal and distal reference diameters (>0.5 mm). This is because, if undersized proximally, malapposition may occur, which could be difficult to correct without risking fracture after deployment. In contrast, if oversized distally, vessel injury around the distal edge may occur if expanded fully, or there could be increased device vessel wall coverage and strut volume in the vessel lumen.42
It has been postulated that the current BVS with bulky struts may require more potent and prolonged antiplatelet therapy secondary to a higher incidence of scaffold thrombosis.43 Therefore, it would be better to avoid using current BVS in patients at high risk of bleeding, planned surgery, and/or who are unlikely to adhere to taking medication. To date, there are little data available regarding BVS for left main trunk lesions, which can be challenging lesion subsets even with DES.44 Therefore, when considering using BVS for left main disease, the operator must carefully evaluate the lesion to treat due to issues of lumen/vessel diameter and the presence of ostial lesions.
IMPLANTING TECHNIQUES
Studies have revealed various success rates with BVS implantations.34 This can be partly attributed to varying operator techniques. In a pooled study containing 2,973 patients treated with the Absorb BVS, only 82.3% of patients were noted to have optimally sized vessels (vessel diameter ≥2.25 mm to ≤3.75 mm). Additionally, only 60.1% underwent pre-dilatation and only 12.4% underwent adequate high-pressure post-dilatation.45 A three-phase preparation, sizing, and post-dilatation (PSP) implantation technique is currently advised in order to achieve maximal effectiveness.46
Phase 1: Preparing the Lesion
The vessel is initially dilated using a noncompliant balloon. The goal is to achieve a pre-dilatation lumen diameter matching the reference diameter (1:1 balloon-to-artery ratio) of the BVS chosen for implantation.
Phase 2: Sizing the Vessel
Prior studies have shown appropriate sizing is of paramount importance in achieving the maximal effectiveness of BVS.34,44 Currently, the Absorb BVS is indicated for coronary artery lesions that are ≤24 mm long and with a reference vessel diameter of ≥2.5 mm and ≤3.75 mm. Due to thick scaffold struts, scaffold overlapping should be minimal, which could predispose the overlapped area to stent thrombosis. Also, BVS have a reduced range of expansion when compared to DES, which should be taken into consideration when sizing the lumen. The use of optical coherence tomography, IVUS, or quantitative coronary analysis is encouraged for optimal placement.
Phase 3: Post Dilatation of the Bioresorbable Vascular Scaffold
The last phase involves high-pressure post-dilation, up to ≤0.5 mm above the nominal scaffold diameter, to ensure the scaffold struts are embedded into the vessel wall. After completing this phase, the goal amount of residual stenosis should be <10%.
The Italian Diffuse/Multivessel Disease ABSORB Prospective Registry (IT-DISAPPEARS) was developed to investigate the procedural and clinical performance of the Absorb BVS in patients with long (>24 mm) single vessel disease and/or multivessel disease.47 In the registry, a pre-specified technique for scaffold implantation was mandated. Indeed, quantitative coronary analysis, optical coherence tomography, or IVUS to assess reference vessel diameter and lesion length, as well as to guide optimal scaffold implantation were recommended. Moreover, high-pressure post-dilatation with non-compliant balloons was also recommended to achieve a residual stenosis ≤10%.47 A total of 2,040 BVS were implanted in 956 patients during the study period. At 1-year follow-up, the rates of all-cause death, non-fatal myocardial infarction, and revascularisation were found to be 1.2%, 5.4%, and 10.9%, respectively. This registry prospective demonstrated that, when a careful technique is used, Absorb BVS implantation can be associated with an excellent safety and efficacy profile, even in patients with high lesion complexity.47 The Absorb IV trial is currently being conducted, which will evaluate the PSP technique prospectively.
COMPARISON OF BIORESORBABLE CORONARY SCAFFOLDS VERSUS DRUG-ELUTING METALLIC STENTS
Table 2 compares the advantages and disadvantages of BRS over drug-eluting metallic stents.
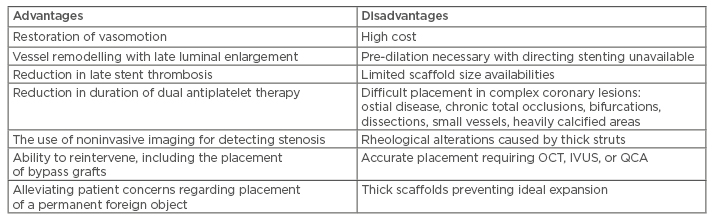
Table 2: Bioresorbable coronary scaffolds: Advantages versus disadvantages over drug-eluting metallic stents.
IVUS: intravascular ultrasound; OCT: optical coherence tomography; QCA: quantitative coronary angiography.
Future Directions
Many new developments are being carried out to improve the shortcomings of older generation BRS. The design of newer generation devices is aimed at producing thinner struts and a smaller crossing profile as compared with the currently available BRS. The new scaffolds under development include the DEsolve, MeRes100 (Meril Life Science, Vapi, India), and Biolute, which have a strut thickness of 100, 100, and 108 μm, respectively.48 This improvement, if successful, will allow proper radial strength to be obtained with a simultaneous decrease in the crossing profile. Additionally, thinner struts might minimise coronary blood flow perturbations and strut protrusion into the vessel lumen resulting in decreased thrombogenicity. Analogous technical improvement can be observed with the Mirage BRS, a microfibre scaffold with streamlined strut geometry and round struts that are supposed to decrease blood flow separation and ensure high shear stress, subsequently reducing platelet activation.49 Another important issue with the BRS is its limited ability to post-dilate (preferably over expand), specifically the Absorb BVS, without fracturing the device. In this regard, the Fantom (a desaminotyrosine-derived polycarbonate scaffold), the DEsolve, and the Fortitude (Amaranth Medical, Mountain View, California, USA) (both PLLA-based polymer scaffolds) have shown greater resistance to overexpansion. In addition to reduced strut thickness, the future iterations for BRS that are currently being worked on include an effective delivery system, complete bioresorption, vessel geometry preservation, vulnerable plaque passivation, vascular physiology, and vasomotor function restoration.
CONCLUSION
In order to overcome the shortcomings of DES, specifically in-stent restenosis and late stent thrombosis, there has been a robust development of BRS. Initial data using BRS demonstrated vasomotor restoration, regression of underlying plaque, and vessel remodelling leading to an increased lumen size. However, the first-generation BRS have demonstrated poor acute performance and increased safety concern, limiting their use in real-world clinical settings. The safety risks associated with Absorb BVS have already resulted in a recent FDA warning to USA physicians, and severe use limitations in Europe, Japan, Australia, and other countries. Newer generation BRS are being developed to overcome the challenges of the first-generation BRS. However, we should not repeat history with BRS, as we did with first-generation DES, without understanding the greater stent thrombosis risk they posed. Research on BVS should be more intense with any new BVS required to undergo proper long-term evaluation in randomised trials versus the best second-generation DES before its widespread clinical adoption.