Abstract
Background: A surrogate classification of breast cancer (BC) molecular subtypes based on immunohistochemistry (IHC) was established at the 13th St. Gallen International Breast Cancer Consensus (SG-BCC). The most controversial point of discussion was the difference between the luminal A and B subtypes. The Ki-67 cut-off that has been used to differentiate these BC subtypes is 14%; however, this cut-off was questioned. This study aimed to identifying the best Ki67 cut-off for determining the luminal BC by PAM50/Prosigna (NanoString Technologies, Seattle, Washington, USA).
Methods: This study included females who were diagnosed with early-stage luminal BC between 2015–2020, and whose samples were subjected to genomic testing using PAM50.
Results: A total of 143 samples were analysed. At the Ki-67 cut-off values of >14%; a correlation of 70.6%, with a sensitivity of 79.1% and a specificity of 55.8%; and a positive predictive value of 75.8% and negative predictive value of 60.4% were observed. When the Ki-67 cut-off was increased to >20%, the percentage of well-classified tumours based on IHC was 76.2%, increasing the agreement by 6.2%. The sensitivity was 93.4%, but the specificity was 46.1%. The positive predictive value was 75.2% while the negative predictive value was 80%, suggesting that IHC has a high probability of diagnosing luminal A and B.
Conclusions: Increasing the Ki-67 cut-off to >20% leads to a better surrogate classification based on IHC and to a higher sensitivity in classifying the luminal subtypes. The authors propose that the cut-off for Ki-67, which is an independent factor, should be globally modified to >20%.
Key Points
1. Advanced techniques such as molecular biology and genome sequencing have altered the way breast cancer is understood. Based on this study, and on subsequent research conducted over the last 20 years, four intrinsic breast cancer subtypes have been characterised: luminal A, luminal B, HER2-enriched, and basal-like subtypes, which differ in terms of incidence, risk factors, prognosis, survival, and sensitivity to treatment.2. The main objective of this study was to determine the optimal cut-off that could differentiate between luminal tumours using Ki-67 as the independent differentiating factor.
3. The study concluded that the specificity and sensitivity that clinicians want to find at the Ki-67 cut-off point depends on the objectives that they want to achieve with the patient. Different clinical factors must guide the clinician, along with the sensitivity and specificity of the Ki-67 cutting method chosen.
BACKGROUND
Over the last few years, advances in molecular biology and genome sequencing have made it possible to identify the genetic alterations that underlie different health conditions, and these advances are particularly important in the field of oncology. Thanks to these advanced techniques, there has been a change in the way a disease as complex and heterogeneous as breast cancer (BC) is understood.1 In 2000, Perou et al.2 established a classification of the intrinsic BC subtypes after analysing the microarrays of complementary DNA of 50 genes.2,3 Based on this study, and on subsequent research conducted over the last 20 years, four intrinsic BC subtypes have been characterised: luminal A, luminal B, HER2-enriched, and basal-like subtypes. These entities significantly differ in terms of incidence, risk factors, prognosis, survival, and sensitivity to treatment.4-6
Perou et al.’s2 working hypothesis was based on the idea that the phenotypic diversity of BC is due to the diversity in its expression patterns, which could be studied with complementary DNA microarrays, leading to the establishment of a new molecular taxonomy for BC. This group analysed 65 surgical breast specimens (including tumours and healthy tissues) obtained from 42 females with locally advanced BC who were treated with neoadjuvant therapy. They identified 496 genes with greater expression variability within the different tumour groups, but with minimum variability between the samples of the same patient (intrinsic genes). At this level of gene expression, the tumours could be grouped into the abovementioned subtypes.2
The luminal subtypes include oestrogen receptor (ER)-positive tumours, with an expression pattern of the luminal epithelium.7-9 These tumours are generally low grade, and less than 20% present TP53 mutations.10 Within the luminal cluster, at least two subtypes exist (luminal A and B), which differ from each other in several respects. The luminal A phenotype exhibits high expression levels of ER and ER-related genes, and low expression levels of HER2 genes and proliferation, including Ki-67. Despite the differences in terms of the number and type of genes analysed in each expression subtype, all results showed that the luminal A subtype has a better prognosis than the luminal B subtype.11 The luminal B subtype accounts for 20% of all BC cases; conversely, it displays a moderate expression of ER and a lower expression of ER-related genes, with variable expression levels of HER2 genes and a higher frequency of TP53 mutations.12 Several studies have suggested that the prognosis and the 10-year survival rate of these tumours are similar to those of the basal-like subtype.13
The HER2 subtype accounts for 10–15% of all BC cases, and it is characterised by high expression levels of HER2 and proliferation genes and low expression levels of ER-related genes.14 In terms of genomic profile, the HER2-positive subtype only refers to tumours that do not express ER. They must not be confused with tumours that are HER2-positive based on immunohistochemistry (IHC) or on a fluorescence in situ hybridisation test or with tumours that express ER, as they are genotypically classified as tumours of the luminal subtype.15
The last subtype is called basal-like because of its similarity to the expression of basal myoepithelial cells in mammary ducts. It represents approximately 15% of all BC cases. It is characterised by the low expression levels of luminal and HER2 genes. This subtype does not correlate with clinical symptoms and is often considered to be a triple-negative phenotype.16-17
Based on the 496 genes analysed in the Perou et al’s2 study, and thanks to the microarray and reverse transcription PCRtechniques, Parker et al.18 simplified the selection of genes in 2009 and reduced it to 45 classifying genes and five control genes, based on which the different intrinsic subtypes were identified. These intrinsic subtypes classified by Perou et al.2 were subsequently ratified by the study led by The Cancer Genome Atlas (TCGA) project, which analysed over 500 BC cases.19 This classification of the intrinsic subtypes has some limitations due to the complex methodology involved. For this reason, it was necessary to develop a classification system for clinical analysis based on the surrogate markers detected via IHC, wherein the different types of BC will be grouped into the abovementioned four main intrinsic subtypes. These IHC surrogate markers are ER, progesterone receptors (PR), HER2, and the proliferation marker Ki-67.
The most commonly used surrogate classification was established in the 13th St. Gallen International Breast Cancer Consensus (SG-BCC), and this classification demonstrates the correlation of survival and prognosis with the intrinsic BC subtypes.20 However, out of the four IHC surrogate markers, Ki-67 has disadvantages; to date, the optimal Ki-67 cut-off that could differentiate luminal A and B tumours is unknown, although a cut-off of 14% is currently accepted. Studies on this subject have used cut-off values ranging from 10–30%.21,22 In other words, there is no fixed value at which the different luminal tumours are distinguished. The clinical guidelines for early-stage BC published by the European Society for Medical Oncology (ESMO) in 2019 proposes a cut-off of 20%.23
Different genetic profiles play an essential role in the identification of intrinsic BC subtypes. Not only do they help determine the intrinsic tumour subtype being analysed, but they also make it possible to assess, through the quantification of specific genes and the use of weighting coefficients, the correct adjuvant therapy used in localised BC with a luminal subtype.24 Several genomic tests used in daily clinical practice have been validated, and four of them (MammaPrint [Agendia, Irvine, California, USA], Oncotype Dx [Genomic Health Redwood City, California, USA], EndoPredict [Myriad Genetics, Salt Lake City, Utah, USA], and PAM50/Prosigna [NanoString Technologies, Seattle, Washington, USA]) are frequently used in the author’s centre.25-28
Based on the studies published by Perou et al. in 2000,2 they later presented a risk predictor based on gene expression in 2009. This predictor could be applied relatively easily in clinical practice and could identify the intrinsic subtypes of BC. This predictor is PAM50, and it measures the expression levels of 50 specifically-selected genes that can be analysed using the RNA obtained from formalin-fixed paraffin-embedded tissues.29 When the ability of PAM50 to identify the four subtypes was compared with the results of molecular classification based on the microarray of RNA of approximately 2,000 genes from fresh tissue, its accuracy was 93%, demonstrating that the test provides information similar to that obtained from 2,000 genes.30
Initially, PAM50 required the use of the genomic quantitative reverse transcription-PCR platform, which allowed for greater clinical applicability; however, the analysis is centralised. In 2001, the nCounter Technology (Nanostring Technologies Inc., Seattle, Washington, USA) made it possible to conduct analyses using degraded RNA without involving amplification, and it can provide results within 72 hours. Moreover, the process is decentralised, meaning tests may be carried out locally. Apart from providing information on the intrinsic subtype of BC, the PAM50 test offers individualised prognostic information based on a predictor known as Risk of Recurrence (ROR),31 which is a score ranging from zero (best prognosis) to 100 points (worse prognosis). This score determines the risk of distance recurrence after 10 years: low (<10% ROR), medium (10–20% ROR), and high risk (>20% ROR).32 Given that this test is not centralised, an analytical validation was conducted in three different laboratories. The results showed that the agreement for the intrinsic subtypes was 97–100% and the standard deviation for the ROR score was 0.67–2.90 points (on a scale of 0–100 points).29 Given the high level of standardisation of the test, it received the 501(k) approval from the U.S. Food and Drug Administration (FDA) in 2013.
Currently, the main indication of the PAM50 test is the prediction of late recurrence of luminal diseases. The prognostic ability of ROR after 5–10 years of monitoring has been retrospectively validated in the ATAC33 and ABCSG-8 studies.34 In both studies, the ROR could identify a group of patients who had a risk of <5% after 5 and 10 years of monitoring. In view of these results, the FDA approved the use of PAM50 in 2014 to predict the risk of late recurrence in the luminal subtypes of BC. The lack of availability of PAM50 in all hospital centres has led to the frequent use of surrogate BC classification via IHC. The possibility of obtaining a better classification of luminal BC cases leads to a better choice of adjuvant therapy and prevents the unnecessary use of chemotherapy (CT) as treatment for low-risk tumours. However, this classification does not seem to clearly distinguish between low- and high-risk tumours.
For this reason, this study aimed to determine the optimal Ki-67 cut-off for surrogate BC classification in order to differentiate luminal tumours based on the correlation between the Ki-67 values obtained via IHC and the intrinsic classification based on PAM50.
METHODS
Inclusion Criteria
The authors carried out a retrospective analysis involving pre- and postmenopausal women who were diagnosed with luminal A or B early-stage BC (N0) via IHC and whose surgical samples were subjected to genomic testing using PAM50. The IHC analysis criteria were based on the 13th St. Gallen Consensus, which was published in 2013. The PAM50 study criteria were approved in 2015 by the Health Department of the Regional Government of Castilla y León: resected breast tumour measuring ≤30 mm without lymph node involvement or micrometastatic disease (N0 or N1mi), ER positive and HER2-negative, which meets at least one and less than two of the following high-risk criteria: histologic grade (Nottingham score) II; Ki-67 >14% and <30%; PRs >20%; weak or moderate expression of ERs (+/++ or ≤50%).
Definition and Analysis of Statistical Values
In this study, sensitivity is defined as the probability that IHC classifies tumours as luminal A when PAM50 classifies them as such, and specificity is defined as the probability that IHC classifies tumours as luminal B when PAM50 classifies them as such. The positive predictive value (PPV) was described as the probability that PAM50 classifies tumours as luminal A when IHC classifies them as such. The negative predictive value (NPV) was defined as the probability that PAM50 classifies tumours as luminal B when IHC classifies them as such.
A concordance analysis between PAM50 and Ki-67 was conducted and the optimal cut-off values for Ki-67 was determined using Cohen’s κ coefficient and receiver operating characteristic (ROC) curve, respectively. The κ concordance was analysed using the criteria established by Ruiz-Morales and Morillo-Zárate in 2004.35 The criterion used to select the cut-off based on the ROC curve was the point closest to the upper-left corner of the curve. The 95% confidence interval of the area under the curve was calculated using the method established by DeLong et al.36 Statistical analysis was conducted using the EZR software (Easy R) v. 1.5.
RESULTS
This study included 143 females with a median age of 54 (32–74) years. The most common histological disease was invasive ductal carcinoma, which was found in 108 patients (75.5%), followed by invasive lobular carcinoma, which was observed in 27 patients (18.9%). Most of the patients (122 out of 143 [85.3%]) had tumours measuring ≤2 cm (pT1) and 21 (14.7%) patients had tumours measuring 2–3 cm long (pT2). Of the patients, 79% (113 out of 143) did not show lymph node involvement, and 21% (30 out of 143) showed micrometastatic disease (pN1mi). All patients had luminal A-like or B-like BC (none of the patients had HER2-enriched BC). Within the 6-year follow-up period, none of the patients (0 out of 143 [0%]) showed recurrence after the adjuvant therapy. The general characteristics of the patients are shown in Table 1.
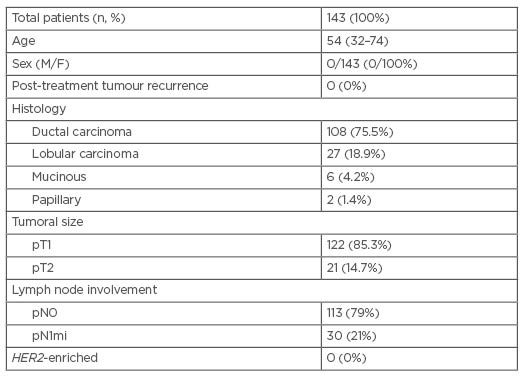
Table 1: General characteristics of the patients included in the study.
F: female; M: male.
In the IHC classification, 64 tumours (44.8%) were classified as luminal A and 79 (55.2%) as luminal B. In the PAM50 analysis, 91 tumours (63.5%) were classified as luminal A and 52 (36.7%) were classified as luminal B. In 41 patients (29%), the therapeutic decision changed after the PAM50 analysis. In the group that received adjuvant hormonal therapy (HT) as the single treatment prior to the test (n=97), CT was added to the treatment in 27 cases (28%). In the group that received CT plus HT prior to the test (n=46), single HT was decided post-test in 14 cases (39%).
Concordance Between Immunohistochemistry and PAM50
The κ coefficient in the analysis of the global concordance between the IHC surrogate classification and the PAM50 classification was 0.16. The analysis of the diagnostic values for the IHC surrogate markers showed that the concordance rate of the correctly classified cases was 57.7. The IHC surrogate classification had a sensitivity of 55.70, a specificity of 60.78%, a PPV of 68.80%, and an NPV of 46.70%.
When the concordance between IHC and PAM50 was analysed for the Ki-67 marker at the current cut-off of 14%, the κ coefficient was 0.35. The percentage of patients that were correctly classified as having luminal A or B tumours based on Ki-67 analysis was 70.63%. The surrogate marker Ki-67 had a sensitivity of 79.1%, a specificity of 55.8%, a PPV of 75.8%, and an NPV of 60.41%. Compared with the standard classification, Ki-67, being an independent marker, provides a better concordance of 23.42% in differentiating luminal A tumours and a reduced accuracy (lower by 5%) in differentiating luminal B tumours.
When the cut-off for Ki-67 as an independent marker was set at 20%, the κ coefficient was 0.44 and the rate of accurate patient classification was 76.22%, which was 5.60% higher than that obtained at the Ki-67 cut-off of 14%. Moreover, the sensitivity was 93.41% and specificity was 46.15% (sensitivity was 14.3% higher and specificity was 9.65% lower than the results obtained at the Ki-67 cut-off of 14%). The abovementioned results are summarised in Table 2.

Table 2: Summary of the statistical values obtained in the differentiation of luminal A and B tumours.
κ: Cohen’s κ coefficient; NPV: negative predictive value; PPV: positive predictive value; PR: progesterone receptor.
The possible influence of PRs on the cut-off point of Ki-67 by IHC was studied. It was observed that the modification of the cut-off points of the PRs in the different points of Ki-67 did not influence the sensitivity and specificity of 14% and 20% in the classification of luminal A and B tumours.
Establishment of the Ki-67 Cut-Off Values with Receiver Operating Characteristic Curve Analysis
The ROC curve for Ki-67, an independent surrogate marker, as determined by IHC is shown in Figure 1. The area under the curve of the ROC curve is 0.78 (95% confidence interval: 0.70–0.85). The Ki-67 cut-off showing the most homogeneous values for sensitivity and specificity was 14% (sensitivity: 79%; specificity: 56%). A Ki-67 cut-off of 10% showed a significant increase in specificity at the expense of a pronounced decrease in sensitivity (specificity: 94%; sensitivity: 43%). The opposite trend was observed when the Ki-67 cut-off was increased to 20%; there was a considerably marked increase in sensitivity with a sharp fall in specificity (sensitivity: 93%; specificity: 46%).
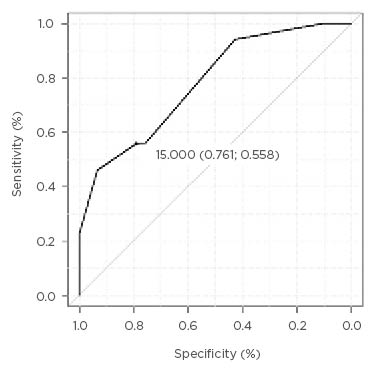
Figure 1: Receiver operating characteristic curve of Ki-67 as an independent diagnostic marker of luminal breast cancer.
The Ki-67 cut-off values of at least 25% demonstrated a 100% sensitivity. By contrast, the specificity was compromised. At a cut-off of 25%, the specificity was 23% and it progressively decreased with the increase in cut-off value. A 100% specificity was reached when the Ki-67 cut-off was 3%, although the shift in threshold from 12% to 10% involved an increase in specificity from 56% to 94% and it entailed a decrease in sensitivity from 76% to 43%.
Clinical Implications of the Ki-67 Cut-Off Values
When the Ki-67 cut-off of 14% was applied in the authors’ sample, the concordance rate between PAM50 and IHC was 70.6%; 91 out of the 143 patients (70.6%) were classified correctly. Within this group, 72 out of the 95 (75.8%) tumours that were classified as luminal A using PAM50 were correctly classified as such in the IHC analysis, whereas 29 out of the 48 (60.4%) tumours classified as luminal B using PAM50 were correctly classified as such in the IHC analysis.
When the Ki-67 cut-off was set at 20%, the concordance rate between IHC and PAM50 was 76.2% (109 out of 143 patients were classified correctly). The percentage of patients who were correctly classified as having luminal A based on IHC and who were later confirmed with PAM50 was 75.2% (85 out of 113). In the case of luminal B tumours, the percentage of tumours that were correctly classified with IHC was 80% (24 out of 30).
In the authors’ sample, the cut-off values below 14% showed reduction in sensitivity, leading to inaccurate classification of luminal A tumours, despite the improvement in the concordance rate for luminal B tumours. Conversely, when the cut-off values were higher than 20%, the increase in sensitivity improved the concordance rate for luminal A tumours. However, the significant loss in specificity entailed the nearly total disappearance of the correct classification of luminal B tumours.
DISCUSSION
In clinical practice, the use of genomic platforms has been essential in the decision-making process in the adjuvant treatment of luminal subtypes of early-stage BC, as well as in the determination of the intrinsic subtypes of BC. The IHC surrogate classification of BC subtypes is a valuable tool when genomic platforms are unavailable. However, the categorisation mechanism of this tool has some shortcomings, particularly in differentiating luminal A and B tumours.
In this study, the authors focused on Ki-67, the most controversial IHC surrogate marker in the literature.37,38 To determine the optimal cut-off that could differentiate between luminal tumours, the authors assessed the concordance between IHC and PAM50 based on the concordance rate (κ coefficient, relative values, and absolute values) and on ROC curve, globally at first. The concordance rate between the subtypes determined via IHC and the intrinsic subtypes determined using PAM50 was 57.7%. This concordance rate is lower than the published ones, such as those reported by Kim et al.39 and Fernández-Martínez et al.40 (61.2% and 70.8%, respectively). The κ coefficient was 0.16, indicating a low concordance between the BC subtype determined via IHC and the true intrinsic subtype. A poor classification of luminal tumours was observed more often in luminal A tumours than in luminal B tumours because, in the authors’ sample, the sensitivity was 5% lower than the specificity. These results indicated that the classification of BC subtypes via IHC could not accurately differentiate luminal breast tumours. The need to improve IHC classification has already been mentioned in other studies.41
The currently accepted cut-off for Ki-67 as an independent diagnostic marker of luminal BC is 14%. However, the 2019 ESMO 2019 clinical practice guidelines for early-stage BC suggest the use of a cut-off of 20% for Ki-67.23 In the authors’ sample, the κ coefficient was 0.35, indicating a low concordance. Thus, luminal tumours are better differentiated when Ki-67 is used as an independent marker than when PR and Ki-67 are considered together, wherein the differentiation between luminal A and B tumours is poorer in patients with luminal BC (positive expression of hormonal ERs and negative expression of HER2). In differentiating luminal tumours, the independent use of Ki-67 provides more accurate results, not only in terms of κ coefficient, but also in terms of the accurate classification of samples (an increase by 12.9%) and in terms of sensitivity (an increase by 23.4% relative to the global classification). In terms of accurate patient classification and sensitivity, the authors found that Ki-67 is a better predictor than IHC surrogate classification for the characterisation of luminal A tumours. The classification of luminal B tumours shows a slightly poorer characterisation derived from a specificity loss of 5%, but this was not reflected in the absolute number of patients in our sample.
This loss of specificity of luminal B tumours is likely to result from a different histological presentation of luminal A tumours. Different genomic or IHC markers that have not been fully characterised at present could make the diagnostic difference between luminal A and B tumours. That is why Ki-67 would be a good marker of IHC for the characterisation of luminal A tumours but not luminal B.
The main objective of this study was to determine the optimal cut-off that could differentiate between luminal tumours using Ki-67 as the independent differentiating factor.21 In the assessment of different cut-off values via ROC curve analysis, the two cut-off values that showed the best results were 14% and 20%. From a global perspective, when the Ki-67 cut-off was increased to 20% in the IHC classification, more tumours were classified correctly as luminal A; however, the question remains whether this would largely affect a poorer characterisation of luminal B tumours. The increase in the cut-off from 14% to 20% increased the concordance rate by nearly 6% (18 out of 143 patients were correctly classified). Also, the κ coefficient increased from 0.35 to 0.44 (from low to moderate concordance for the differentiation of luminal tumours). This improvement in the concordance rate was accompanied by a significant increase in sensitivity (by over 14%) and in the NPV (by nearly 20%). The 8% decrease in specificity explains the poor classification of luminal B tumours. In the authors’ sample, 5 out of the 143 patients (3.5%) were poorly classified. Considering these results, the increase in the Ki-67 cut-off from 14% to 20% led to a better classification of luminal A tumours at the expense of a slightly worse classification of luminal B tumours; however, it does not affect the κ coefficient or the concordance rate.
It is important to note that the proposed new classification, with the elevation of the cut-off point from 14% to 20%, is one more diagnostic tool that helps define patients in luminal subtype A or B. There is a small percentage of patients with luminal subtype B who could be misclassified, so it is important to assess the patient to make a decision on the use of CT adjuvant in these patients. Age, menstrual status, or the existence of comorbidities in conjunction with the values of immunohistochemistry should be used to help to define what type of complementary treatment patients should receive.
The specificity and sensitivity that clinicians want to find at the Ki-67 cut-off point depends on the objectives that they want to achieve with the patient. Different clinical factors must guide the clinician, along with the sensitivity and specificity of the Ki-67 cutting method chosen. Therefore, it is key to bear in mind that in patients who, due to clinical or tumour factors, might have had a high risk or recurrence, the Ki-67 cut-off point could decrease to 14%, if necessary. Considering these factors, if there are no specific indications that point towards a cut-off point, assessing the specificity and sensitivity of Ki-67 the most optimal cut-off point would be 20%.
The authors’ data suggest that it is advisable to increase the Ki-67 cut-off from 14% to 20% in the IHC surrogate classification of BC. However, it is important to consider the sample size. Further studies are needed, given that a larger sample size may validate whether the improved classification of luminal A tumours do not influence the characterisation of luminal B tumours, as seen in the authors’ results.
In conclusion, based on the present results, the Ki-67 cut-off at 14% presents the most homogeneous sensitivity and specificity for the classification of luminal A and B subtypes of BC. However, a cut-off value of 20% provides a better classification of luminal tumours because of its higher sensitivity, which leads to a better diagnosis of patients with luminal A tumours. Increasing the Ki-67 cut-off to 20% to differentiate between luminal tumours may prevent unnecessary CT treatments. New studies involving a larger sample are warranted for a prospective validation of this cut-off in order to determine the optimal Ki-67 cut-off.