Abstract
The relation between pneumonia and influenza is regularly addressed in modern music. Epidemiological data obtained during influenza pandemics, as well as during seasonal influenza, illustrate and underscore this association. Even though the number of pneumonia cases are generally under-reported and blood tests show a lack of sensitivity, a clear link between influenza and pneumonia can still be observed. In fact, the majority of mortality during influenza pandemics is due to pneumonia caused by a bacterial superinfection, in most cases Streptococcus pneumoniae. Vaccination is a powerful tool to prevent the development of both influenza and pneumonia in children, as well as in the elderly. Cellular and molecular data show that influenza can lead to changes in the integrity of lung epithelial cells, including desialysation of carbohydrate moieties, which favour attachment and invasion of S. pneumoniae. Further elucidation of these mechanisms could lead to targeted intervention strategies, in which universal influenza vaccines could play a role.
INTRODUCTION
The epidemiological association between influenza and pneumonia is well known. In this paper, the authors review the cellular and molecular mechanisms that underlie this association.
The association between influenza and pneumonia is also well known to the general public, as reflected in pop culture. Previously, the authors have analysed the context in which pneumonia (the disease) is dealt with in rock songs.1 One of the findings was that most songwriters and singers have little idea about the infectious nature of pneumonia.2 Taking into account that the causes of pneumonia, as well as its symptoms, treatment, and prognosis, are poorly described in songs, it is remarkable that the association between pneumonia and influenza is rather adequately depicted in music.1 The best example may be from Huey ‘Piano’ Smith: “Young man rhythm’s got a hold of me too, I got the rockin’ pneumonia and the boogie woogie flu,” from the 1957 record “Rockin’ Pneumonia and the Boogie Woogie Flu”. Followed later in 1960, The Genies released their song “Twisting Pneumonia”: “I’ve got the twistin’ pneumonia, the shimmy shimmy flu, the doctor is gonna tell you that there ain’t no cure.” Because the relationship between fantasy forms of pneumonia (rockin’, twistin’) and fantasy forms of influenza (boogie woogie, shimmy shimmy) is so evidently described in music, the epidemiological and molecular mechanisms underlying this relation warrant closer analysis. Hopefully this will lead to a moment in time when ‘the doctor is gonna tell you that there is a cure’.
THE EPIDEMIOLOGICAL ASSOCIATION BETWEEN INFLUENZA AND PNEUMONI
In the 20th century, the world experienced three major influenza pandemics. Namely, the Spanish influenza in 1918 (H1N1), Asian influenza in 1957 (H2N2), and Hong Kong influenza in 1968 (H3N2). The 1918 pandemic had an especially large impact, with conservative estimates of 40–50 million deaths following H1N1 infection.3 Many studies have shown that bacterial superinfection causing pneumonia following an influenza infection leads to severe disease and mortality. It has been estimated that >95% of deaths during the 1918 pandemic were complicated by bacterial pneumonia, most commonly by Streptococcus pneumoniae.4 However, other common respiratory pathogens, including Staphylococcus aureus, Haemophilus influenzae, and Streptococcus pyogenes were all identified as predominant pathogens in various individual studies.5 It has been predicted that due to the ever-increasing world population and global mobility, both work related and recreational, a future influenza outbreak with a strain with similar virulence to the 1918 Spanish influenza strain may cause even higher mortality rates, with an estimate of between 50 and 80 million deaths.6 Such an outbreak would primarily affect developing countries, because of the limited preparedness and availability of healthcare facilities. Additionally, an epidemiological risk factor is the higher carriage rates of S. pneumoniae among children, as well as adults, in combination with limited availability of pneumococcal conjugate vaccine and antibiotics.7 Generally, people living in sub-Saharan countries experience the highest rates of influenza-associated respiratory deaths, whereas eastern Mediterranean and southeast Asian countries have lower, but still high, death rates.8,9 These findings underline the importance of understanding the synergy and copathogenesis between influenza and subsequent infection by bacterial pathogens, leading to bacterial pneumonia.
An influenza outbreak does not have to be a pandemic to cause substantial mortality. Annual seasonal influenza episodes are associated with significant morbidity. The Centre for Disease Control and Prevention (CDC) estimates that there are between 3 and 5 million annual cases of severe illness due to influenza, and between 291,000 and 646,000 deaths that are a direct consequence of seasonal influenza-related respiratory illnesses.9 Children and the elderly are especially susceptible, particularly those who are <4 years and >65 years of age. Children <1 year of age account for >50% of all influenza-associated deaths in young children.10 Influenza numbers reach their peak during the winter months. The incidence is highest during this time of year because the aerosol spread of the influenza virus depends on both the humidity and temperature of the air. Both cold and dry conditions favour transmission; thus,11 the environmental conditions favouring influenza transmission are relatively well known.12-14 For S. pneumoniae, the transmission mechanisms are known but not the environmental conditions.
Seasonal outbreaks of influenza, rather than pandemics, also are associated with a higher incidence of pneumonia. In the Netherlands, a clear rise in pneumonia can be observed during the peak of the influenza season (Figure 1).15
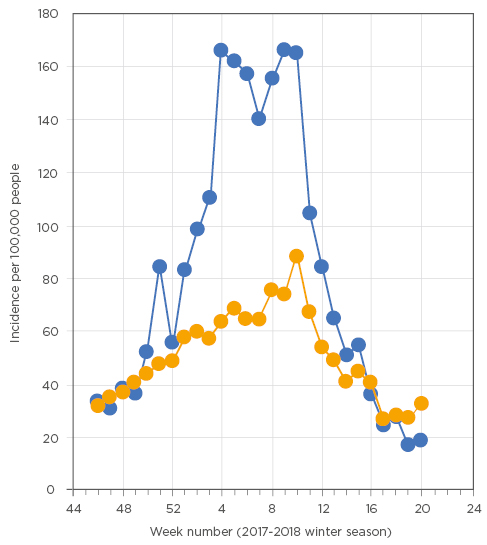
Figure 1: Seasonal trends in incidence of influenza-like illness (blue line) and pneumonia (orange line) as reported by general practitioners in the Netherlands during the 2017-18 winter season.
Data derived from the public available weekly surveillance reports published by NIVEL.15
However, influenza-related pneumonia is most likely highly under-reported, because in cases of community-acquired pneumonia there is often no culture made of the disease-inducing bacterium. Furthermore, blood cultures in patients with pneumonia lack sensitivity, providing accurate results in only 10–15% of cases. However, even when including sputum cultures, urinary tests, and serotype specific antibodies responses, the aetiological agent responsible for community acquired pneumonia often cannot be established.16-18
Pneumonia poses a significant risk for morbidity and mortality in young children as well as the elderly. In 2008, approximately 156 million children worldwide were affected by pneumonia, with an approximate 1.3 million deaths, accounting for 18% of all deaths of children <5 years old.19,20 Furthermore, in people >65 years of age, 40% of pneumonia cases are of a severity that requires hospital admission.21
Vaccination is a primary strategy to reduce the burden imposed by influenza. Currently, the CDC recommends the annual influenza vaccine for everyone ≥6 months of age.9 Live-attenuated influenza vaccines were shown to be most effective in those between 6 months and 7 years of age.22 The influenza vaccine’s efficacy is 56% (95% confidence interval [CI]: 39–68%) for preventing respiratory illness, 53% (CI: 35–66%) for preventing pneumonia, 50% (CI: 28–65%) for preventing hospitalisation, and 68% (CI: 56–76%) for preventing death.23 Seasonal influenza vaccination thus shows efficacy in reducing pneumonia morbidity and mortality.
Following the introduction of pneumococcal conjugate vaccination of children in the USA and elsewhere, the incidence of clinically diagnosed all-cause pneumonia declined by 39% (95% CI: 22–52%).24 Additionally, pneumococcal conjugate vaccines have played an important role in reducing influenza-associated morbidity, preventing 31% (95% CI: 15–43%) of pneumonia cases associated with any of seven respiratory viruses in hospitalised children.25 It was also shown that the children that received the vaccine and subsequently developed laboratory-confirmed influenza were at a 45% lower risk (95% CI: 14–64%) of hospitalisation due to the influenza-associated pneumonia.26 It would be highly unlikely that vaccination with pneumococcal conjugate vaccines (composed of pneumococcal polysaccharides conjugated to bacterial carrier proteins) would have a direct protective effect against infection with respiratory viruses. Therefore, the interpretation of these data would be that recipients infected with respiratory viruses had a lower subsequent complication rate of pneumococcal infection, due to serotype-specific protection. These children, protected against superimposed pneumococcal pneumonia developing due to the viral (influenza) infection, were less likely to progress to pneumonia requiring hospitalisation.27
In elderly people, influenza vaccination lowers the risk of laboratory-confirmed influenza.28 Additional research in immunocompetent individuals aged ≥65 years examined the effects of influenza and pneumococcal vaccination in the prevention of hospital admissions. The influenza vaccine has an efficacy of 30% (95% CI: 17–42%) for high-risk and a 40% (95% CI: 1–64%) efficacy for median-risk elderly persons in preventing influenza and pneumonia hospitalisations and that the pneumococcal vaccine has a 75% (95% CI: 57–85%) efficacy in preventing invasive pneumococcal disease in people >65 years of age.29,30 These studies suggest that the vaccination of both infants and the elderly may greatly reduce influenza-related morbidity and, combined with the data on herd immunity, suggest that immunisation of children may additionally protect adults as well.26 This has been demonstrated both for influenza31 as well as pneumococcal vaccination;32 in both instances, childhood vaccination had an indirect protective effect on the elderly.
CELLULAR AND MOLECULAR MECHANISMS UNDERLYING THE INTERACTION BETWEEN INFLUENZA AND PNEUMONIA
As indicated and discussed above, during and after the influenza pandemic in 1918, the association of a respiratory virus predisposing to a bacterial infection was already recognised.33 Multiple mechanisms could explain this association between influenza and pneumococcal infections, including impairment of host immune responsiveness, exacerbation of inflammatory mediators, and biofilm dispersion. The influenza A virus impairs both the host’s innate and adaptive immune systems by downregulating IFN-γ production and by other means.34 Infection with influenza H1N1 leads to a decrease in CD4+ T lymphocytes and B lymphocytes.35 Furthermore, viral infections of the airways can induce a ‘cytokine storm’ and the production of various other mediators, including histamine and leukotriene E4, that damage local lung tissue indirectly;36-38 thus, influenza infected patients would become more susceptible to diseases such as pneumonia. In the nasopharynx of patients already colonised, pneumococci have formed biofilms. Influenza virus infection can trigger biofilm dispersion and active release of pneumococci.39 It has been observed, both in vitro and in vivo, that during influenza infection, pneumococcal colonisation of respiratory epithelial surfaces can increase. In vitro data indicate that desialylation of airway epithelial cells, which occurs during influenza virus infection, can enhance pneumococcal adhesion via galectin binding.40 Influenza-virus-infected mice failed to kill S. pneumoniae in vivo.41 Additionally, similar relationships have been demonstrated in other viral respiratory viruses and bacteria that can cause pneumonia in humans. One of the first studies in that respect was conducted by Sanford et al.42 by means of an adherence assay. In this experiment, group B Streptococcus and several other streptococcal species were found colonising only on the cells infected with influenza virus. A more recent study showed that cells infected with swine influenza virus have enhanced bacterial adherence and invasion of deeper tissues of Streptococcus suis,43 a bacterium that can also cause pneumonia in humans.44 Evidence has shown that viruses might promote bacterial infection by facilitating the colonisation, adherence, and translocation of bacteria through the epithelium. There are a number of mechanisms determining these processes, which involve changes and damage to host epithelial cells, several specific viral virulence determinants, and extracellular molecules, which are detailed below.
Viruses Damage Host Respiratory Epithelial Cells Facilitating Bacterial Adhesion
Infection with influenza virus results in multiple changes in the lung epithelial cells. Upon infection, inflammatory reactions are initiated, aiming to neutralise the virus but at the same time inevitably resulting in damage to the epithelial cells that mediate respiratory gas exchange. As the respiratory mucosa (the physical and chemical barrier to infection by producing mucus, surfactants, and other inflammatory mediators) is damaged, opportunistic bacteria, such as S. pneumoniae, can more easily invade and colonise the lung tissue.45 The deposition of dead cells and cell fragments into the airways also provides a rich source of nutrients for bacterial growth.46 Furthermore, cilia are damaged and ciliary motion becomes unco-ordinated, causing impairment of their cleaning function.47 Both the respiratory epithelial damage and impairment in protective mechanism caused by (influenza) viruses facilitates the adherence of bacteria to host cells. Evidence for this mechanism was reported in pathologic examination of patients who died of community acquired S. aureus pneumonia in the 1957 influenza pandemic. Autopsies showed that Staphylococci were adhered in regions of the tracheobronchial tree where the epithelial layer had been destroyed and sloughed off.48 However, most seasonal influenza strains do not result in severe lung damage but can still facilitate bacterial superinfection.49
VIRULENCE DETERMINANTS OF INFLUENZA INCREASE RECEPTOR AVAILABILITY FOR BACTERIA
While viruses expose sites for attachment as previously outlined, bacteria also possess a wide range of virulence factors, such as pneumococcal surface protein A, choline-binding protein A, and pneumococcal serine-rich repeat protein.50 These factors can be used for adherence to the basement membrane or extracellular matrix constituents of the host, such as fibrin, fibrinogen, and collagen.51 S. pneumoniae illustrates this point clearly, as it was found to bind strongly to fibronectin. Viral infection also upregulates expression of fibronectin. Bacterial binding was thus enhanced proportionally to the increase of fibronectin and duration of exposure.52 Moreover, influenza viruses can increase viral fitness and cytotoxic potential by antigenic variation and mutations, thus enhancing epithelial damage and subsequently facilitating bacterial infection.53,54
In addition to changing their antigenic surface, influenza infection can increase receptor availability for bacterial adherence and infection through three other mechanisms. First, influenza viruses insert their glycoproteins, neuraminidase and haemagglutinin, into the host membrane, which subsequently acts as a potential receptor for bacteria.55 Furthermore, viral neuraminidase cleaves sialic acid residues, including the sialic that cover bacterial receptors on cell surface carbohydrates, thus promoting bacterial adherence and invasion.56 Indeed, it was found that treatment with neuraminidase inhibitor (oseltamivir) was associated with a reduction in mortality of at-risk patients diagnosed with the influenza A H1N1pdm09 virus infection.57 In fact, this type of treatment was also widely used during the 2009–2010 influenza A H1N1 pandemic. However, neuraminidase and haemagglutinin inhibitors only inhibit streptococcal adherence to influenza-infected cells, not of Staphylococci, suggesting the presence of other cellular receptors for bacteria.
A second mechanism shows how viral infections can contribute to bacterial superinfection. The virus triggers a host inflammatory response, altering the cell membrane, and thus contributes to bacterial adherence. Influenza virus infection upregulated platelet activating factor receptor expression in lung epithelia, which promotes adhesion of S. pneumoniae.58 Other respiratory viruses, such as respiratory syncytial virus, upregulate receptors that bacteria can bind to, such as CD14, CD15, and CD18, and receptors mediated by outer membrane protein P5 homologous fimbriae.59,60 It is interesting to note that cigarette smoke and e-cigarette vapour both increase the expression of platelet activating factor receptor.61,62
A third and final mechanism, more general in nature, is that areas of incomplete wound healing (induced by influenza or otherwise) might be avid binding sites for bacteria. Fibrinogen expression is increased in incomplete wound healing.62 The surface adhesin PfbA of S. pneumoniae binds to fibrinogen.64 Furthermore, bacteria, including S. pneumoniae, can also adhere to extracellular matrix components involved in tissue repair, including laminin and type I and type IV collagen.5
CONCLUSION
The authors have elaborated on the special interaction between (a rocking, or whatever cause) pneumonia and the (boogie woogie) flu. There are arguments to propose that other respiratory viruses can pave the airways for bacterial pneumonia.25,27 As indicated above, in a substantial number of patients with community-acquired pneumonia, a causative agent has not been found, so these could also be pneumococcal pneumonia.17,18 In cases where influenza virus, or any other respiratory virus for that matter, is found, it can be debated whether this infection would be sufficient argument for the classification of viral pneumonia.
The interaction between influenza, other respiratory viruses, and S. pneumoniae is complex and based on multiple mechanisms. Full understanding of these mechanisms, as well as their mutual interplay, is relevant for a better estimation of the burden of pneumonia in young children. Nasopharyngeal carriage with pneumococci is high during the first 3–5 years of age;65,66 mucosal pneumococcal infections, such as otitis media, are prevalent during this period. Fortunately, most children do not develop a pneumococcal pneumonia. The triggering event for this development could be influenza infection. From such a perspective, it could be speculated that universal influenza vaccines could offer the broadest possible coverage against pneumococcal pneumonia in young children.67,68 The moment these vaccines become available, clinical studies should include their effect on pneumococcal pneumonia.